Describe critical components of groundwater
What You’ll Learn to Do
- Differentiate between groundwater and the water table
- Describe how groundwater is affected by porosity and permeability of geologic materials with respect to different types of aquifers.
- Compare and contrast potential well use within various types of aquifers.
- Identify issues with groundwater withdrawal
- Describe issues with the quality of groundwater and keeping water clean
Groundwater
Although this may seem surprising, water beneath the ground is commonplace. Usually groundwater travels slowly and silently beneath the surface, but in some locations it bubbles to the surface at springs. The products of erosion and deposition by groundwater were described in the Erosion and Deposition chapter.
Groundwater is the largest reservoir of liquid fresh water on Earth and is found in aquifers, porous rock and sediment with water in between. Water is attracted to the soil particles and capillary action, which describes how water moves through a porous media, moves water from wet soil to dry areas.
Aquifers are found at different depths. Some are just below the surface and some are found much deeper below the land surface. A region may have more than one aquifer beneath it and even most deserts are above aquifers. The source region for an aquifer beneath a desert is likely to be far from where the aquifer is located; for example, it may be in a mountain area.
The amount of water that is available to enter groundwater in a region is influenced by the local climate, the slope of the land, the type of rock found at the surface, the vegetation cover, land use in the area, and water retention, which is the amount of water that remains in the ground. More water goes into the ground where there is a lot of rain, flat land, porous rock, exposed soil, and where water is not already filling the soil and rock.
The residence time of water in a groundwater aquifer can be from minutes to thousands of years. Groundwater is often called “fossil water” because it has remained in the ground for so long, often since the end of the ice ages.
Aquifers
Features of an Aquifer
To be a good aquifer, the rock in the aquifer must have good:
- porosity: small spaces between grains
- permeability: connections between pores
This animation shows porosity and permeability. The water droplets are found in the pores between the sediment grains, which is porosity. When the water can travel between ores, that’s permeability.
To reach an aquifer, surface water infiltrates downward into the ground through tiny spaces or pores in the rock. The water travels down through the permeable rock until it reaches a layer that does not have pores; this rock is impermeable (figure 1). This impermeable rock layer forms the base of the aquifer. The upper surface where the groundwater reaches is the water table.
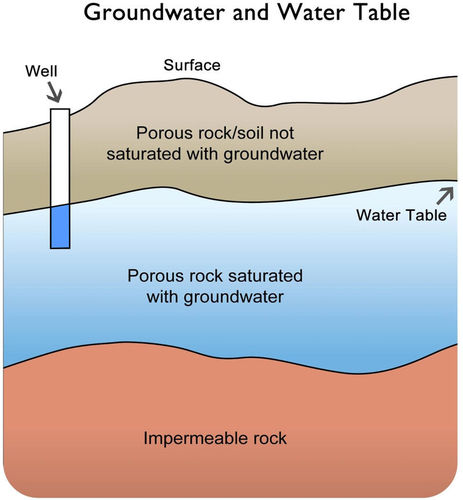
Figure 1. Groundwater is found beneath the solid surface. Notice that the water table roughly mirrors the slope of the land’s surface. A well penetrates the water table.
The Water Table
For a groundwater aquifer to contain the same amount of water, the amount of recharge must equal the amount of discharge. What are the likely sources of recharge? What are the likely sources of discharge?
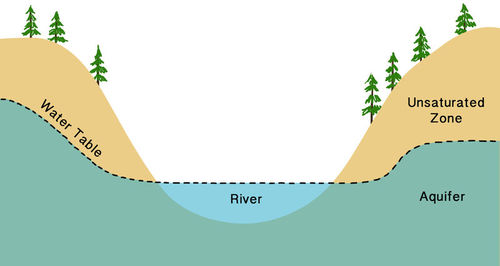
Figure 2. The top of the stream is the top of the water table. The stream feeds the aquifer.
In wet regions, streams are fed by groundwater; the surface of the stream is the top of the water table (figure 2). In dry regions, water seeps down from the stream into the aquifer. These streams are often dry much of the year. Water leaves a groundwater reservoir in streams or springs. People take water from aquifers, too.
What happens to the water table when there is a lot of rainfall? What happens when there is a drought? Although groundwater levels do not rise and fall as rapidly as at the surface, over time the water table will rise during wet periods and fall during droughts.
One of the most interesting, but extremely atypical types of aquifers is found in Florida. Although aquifers are very rarely underground rivers, in Florida water has dissolved the limestone so that streams travel underground and above ground (figure 3).
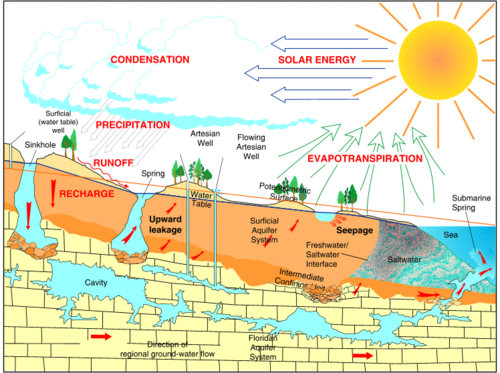
Figure 3. In Florida, groundwater is sometimes not underground.
Porosity and Permeability

Figure 4. A spring coming out of the shale near Red Creek. Yes, that water is black! (Photo: Matt Herod)
As we’ve learned, groundwater is simply water that exists underground. However, there are still lots of misconceptions about how people envision groundwater. Many envision large underground lakes and rivers, and while those do exist, they represent an infinitesimally small percentage of all groundwater. Generally speaking groundwater exists in the pore spaces between grains of soil and rocks. Imagine a water filled sponge. All of the holes in that sponge are water-filled. By squeezing that sponge we force the water out, similarly, by pumping an aquifer we force the water out of pore spaces.
There are lots of terms in hydrogeology, most of which are very simple, but essential. Here are a few of the big ones and their meanings.
Porosity
Porosity is an intrinsic property of every material. It refers to the amount of empty space within a given material. In a soil or rock the porosity (empty space) exists between the grains of minerals. In a material like gravel the grains are large and there is lots of empty space between them since they don’t fit together very well. However, in a material like a gravel, sand and clay mixture the porosity is much less as the smaller grains fill the spaces. The amount of water a material can hold is directly related to the porosity since water will try and fill the empty spaces in a material. We measure porosity by the percentage of empty space that exists within a particular porous media.
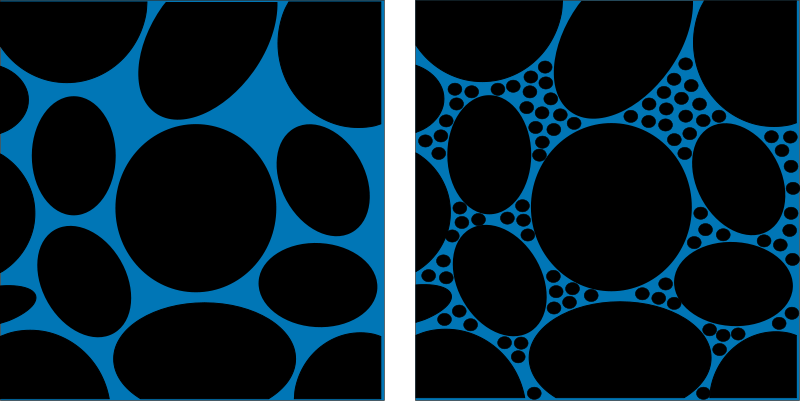
Figure 5. Porosity in two different media. The image on the left is analagous to gravel whereas on the right smaller particles are filling some of the pores and displacing water. Therefore, the water content of the material on the right is less. (Source: Wikipedia)
Permeability
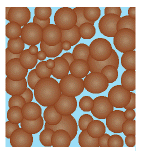
Figure 6. Video showing how connected pores have high permeability and can transport water easily. Note that some pores are isolated and cannot transport water trapped within them.
Permeability is another intrinsic property of all materials and is closely related to porosity. Permeability refers to how connected pore spaces are to one another. If the material has high permeability than pore spaces are connected to one another allowing water to flow from one to another, however, if there is low permeability then the pore spaces are isolated and water is trapped within them. For example, in a gravel all of the pores well connected one another allowing water to flow through it, however, in a clay most of the pore spaces are blocked, meaning water cannot flow through it easily.
Aquifer
An aquifer is a term for a type of soil or rock that can hold and transfer water that is completely saturated with water. That means that all it is simply a layer of soil or rock that has a reasonably high porosity and permeability that allows it to contain water and transfer it from pore to pore relatively quickly and all of the pore spaces are filled with water. Good examples of aquifers are glacial till or sandy soils which have both high porosity and high permeability. Aquifers allows us to recover groundwater by pumping quickly and easily. However, overpumping can easily reduce the amount of water in an aquifer and cause it to dry up. Aquifers are replenished when surface water infiltrates through the ground and refills the pore spaces in the aquifer. This process is called recharge. It is especially important to ensure that recharge is clean and uncontaminated or the entire aquifer could become polluted. There are two main types of aquifer. An unconfined aquifer is one that does not have an aquitard above it but usually does below it.
When a water-bearing rock readily transmits water to wells and springs, it is called an aquifer. Wells can be drilled into the aquifers and water can be pumped out. Precipitation eventually adds water (recharge) into the porous rock of the aquifer. The rate of recharge is not the same for all aquifers, though, and that must be considered when pumping water from a well. Pumping too much water too fast draws down the water in the aquifer and eventually causes a well to yield less and less water and even run dry. In fact, pumping your well too fast can even cause your neighbor’s well to run dry if you both are pumping from the same aquifer.
In the diagram below, you can see how the ground below the water table (the blue area) is saturated with water. The “unsaturated zone” above the water table (the greenish area) still contains water (after all, plants’ roots live in this area), but it is not totally saturated with water. You can see this in the two drawings at the bottom of the diagram, which show a close-up of how water is stored in between underground rock particles.
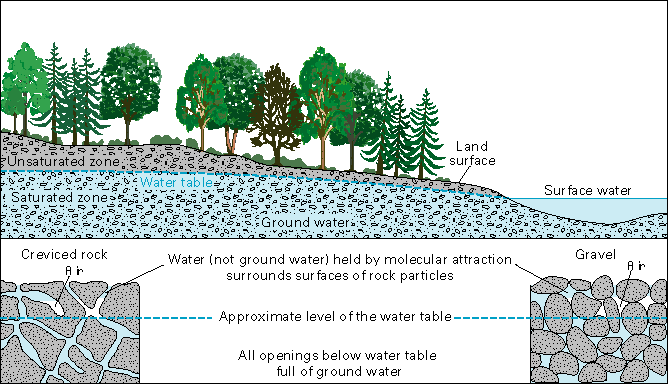
Figure 7.
Sometimes the porous rock layers become tilted in the earth. There might be a confining layer of less porous rock both above and below the porous layer. This is an example of a confined aquifer. In this case, the rocks surrounding the aquifer confines the pressure in the porous rock and its water. If a well is drilled into this “pressurized” aquifer, the internal pressure might (depending on the ability of the rock to transport water) be enough to push the water up the well and up to the surface without the aid of a pump, sometimes completely out of the well. This type of well is called artesian. The pressure of water from an artesian well can be quite dramatic.
A relationship does not necessarily exist between the water-bearing capacity of rocks and the depth at which they are found. A very dense granite that will yield little or no water to a well may be exposed at the land surface. Conversely, a porous sandstone, such as the Dakota Sandstone mentioned previously, may lie hundreds or thousands of feet below the land surface and may yield hundreds of gallons per minute of water. Rocks that yield freshwater have been found at depths of more than 6,000 feet, and salty water has come from oil wells at depths of more than 30,000 feet. On the average, however, the porosity and permeability of rocks decrease as their depth below land surface increases; the pores and cracks in rocks at great depths are closed or greatly reduced in size because of the weight of overlying rocks.
Water Movement in Aquifers
Water movement in aquifers is highly dependent of the permeability of the aquifer material. Permeable material contains interconnected cracks or spaces that are both numerous enough and large enough to allow water to move freely. In some permeable materials groundwater may move several metres in a day; in other places, it moves only a few centimeters in a century. Groundwater moves very slowly through relatively impermeable materials such as clay and shale.
After entering an aquifer, water moves slowly toward lower lying places and eventually is discharged from the aquifer from springs, seeps into streams, or is withdrawn from the ground by wells. Groundwater in aquifers between layers of poorly permeable rock, such as clay or shale, may be confined under pressure. If such a confined aquifer is tapped by a well, water will rise above the top of the aquifer and may even flow from the well onto the land surface. Water confined in this way is said to be under artesian pressure, and the aquifer is called an artesian aquifer.
Visualizing Artesian Pressure
Here’s a little experiment to show you how artesian pressure works. Fill a plastic sandwich baggie with water, put a straw in through the opening, tape the opening around the straw closed, do not point the straw towards your teacher or parents, and then squeeze the baggie. Artesian water is pushed out through the straw.
Aquitard
The other type is a confined aquifer that has an aquitard above and below it. An aquitard is basically the opposite of an aquifer with one key exception. Aquitards have very low permeability and do not transfer water well at all. In fact, in the ground they often act as a barrier to water flow and separate two aquifers. The one key exception is that aquitards can have high porosity and hold lots of water however, due to the their low permeability they are unable to transmit it from pore to pore and therefore water cannot flow within an aquitard very well. A good example of an aquitard is a layer of clay. Clay often has high porosity but almost no permeability meaning it is essentially a barrier which water cannot flow through and the water within it is trapped. However, there is still limited water flow within aquitards due to other processes that I won’t get into now.
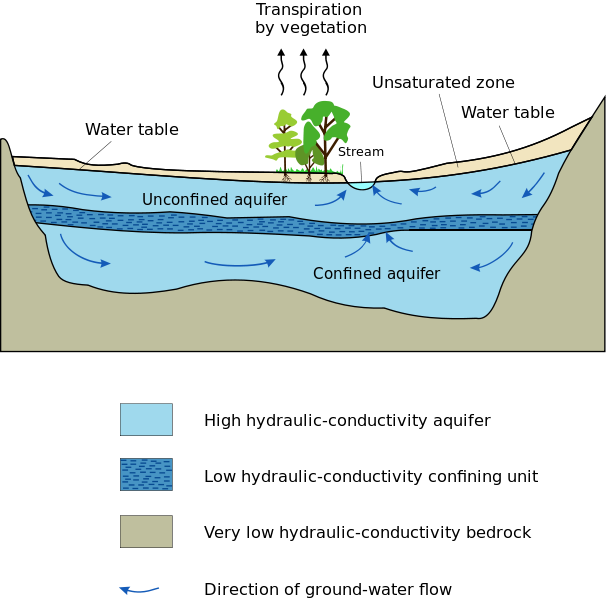
Figure 8.
Aquifers and Well-Potential
There’s a good chance that the average Joe who had to dig a well in ancient Egypt probably did the work with his hands, a shovel, and a bucket. He would have kept digging until he reached the water table and water filled the bottom of the hole. Some wells are still dug by hand today, but more modern methods are available. It’s still a dirty job, though!
Wells are extremely important to all societies. In many places wells provide a reliable and ample supply of water for home uses, irrigation, and industries. Where surface water is scarce, such as in deserts,people couldn’t survive and thrive without groundwater.
Types of Wells
Digging a well by hand is becoming outdated today (would YOU want to do it?). Modern wells are more often drilled by a truck-mounted drill rig. Still, there are many ways to put in a well—here are some of the common methods.
Dug Wells
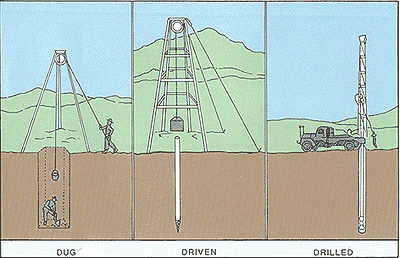
Figure 9. Well Types
Hacking at the ground with a pick and shovel is one way to dig a well. If the ground is soft and the water table is shallow,then dug wells can work. Historically, dug wells were excavated by hand shovel to below the water table until incoming water exceeded the digger’s bailing rate . The well was lined with stones, brick, tile, or other material to prevent collapse, and was covered with a cap of wood, stone, or concrete. They cannot be dug much deeper than the water table — just as you cannot dig a hole very deep when you are at the beach… it keeps filling up with water!
Driven Wells
Driven wells are still common today. They are built by driving a small-diameter pipe into soft earth, such as sand or gravel. A screen is usually attached to the bottom of the pipe to filter out sand and other particles. Problems? They can only tap shallow water, and because the source of the water is so close to the surface, contamination from surface pollutants can occur.
Drilled Wells
Most modern wells are drilled, which requires a fairly complicated and expensive drill rig. Drill rigs are often mounted on big trucks. They use rotary drill bits that chew away at the rock, percussion bits that smash the rock, or, if the ground is soft,large auger bits. Drilled wells can be drilled more than 1,000 feet deep. Often a pump is placed at the bottom to push water up to the surface.
Water Levels in Wells
Ground-water users would find life easier if the water level in the aquifer that supplied their well always stayed the same. Seasonal variations in rainfall and the occasional drought affect the “height” of the underground water level. If a well is pumped at a faster rate than the aquifer around it is recharged by precipitation or other underground flow, then water levels around the well can be lowered. The water level in a well can also be lowered if other wells near it are withdrawing too much water. When water levels drop below the levels of the pump intakes, then wells will begin to pump air—they will “go dry.”
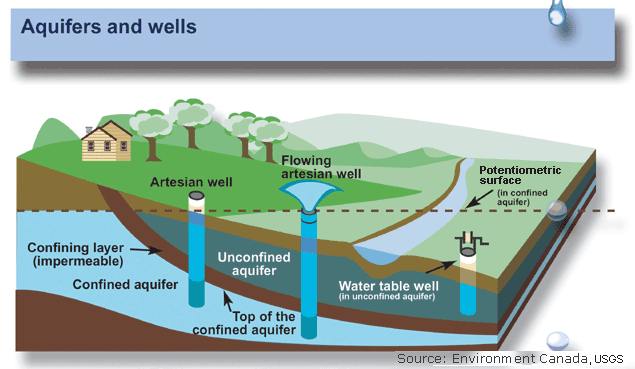
Figure 10. Aquifers and Wells
Groundwater Withdrawal
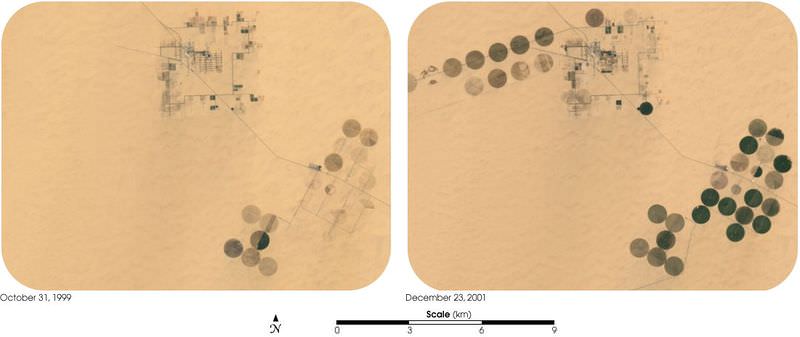
Figure 11
Is it good to make the desert bloom?
Many sunny, arid regions are good for growing crops as long as water can be added. Some of the increase in productivity is due to farming in regions that are technically too dry. Groundwater can be used to make the desert bloom, but at what cost? And for how long? Eventually the wells will run dry.
Groundwater Overuse
Some aquifers are overused; people pump out more water than is replaced. As the water is pumped out, the water table slowly falls, requiring wells to be dug deeper, which takes more money and energy. Wells may go completely dry if they are not deep enough to reach into the lowered water table.
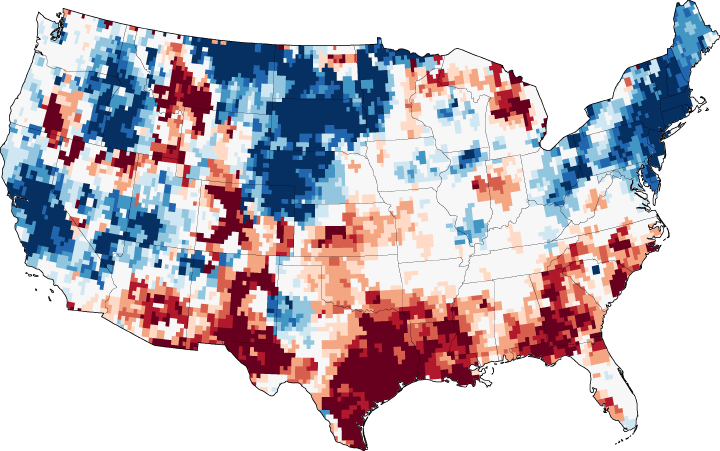
Figure 12. Intense drought has reduced groundwater levels in the southern U.S., particularly in Texas and New Mexico.
Other problems may stem from groundwater overuse. Subsidence and saltwater intrusion are two of them.
Ogallala Aquifer
The Ogallala Aquifer supplies about one-third of the irrigation water in the United States. The Ogallala Aquifer is widely used by people for municipal and agricultural needs. (Figure 13). The aquifer is found from 30 to 100 meters deep over an area of about 440,000 square kilometers!
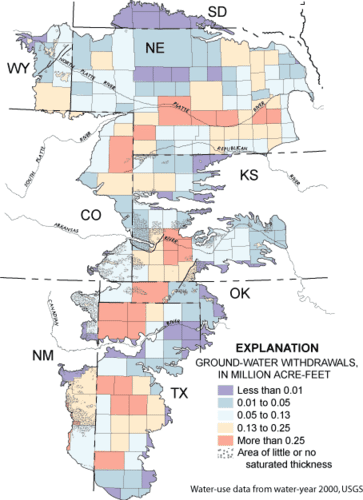
Figure 13. The Ogallala Aquifer is found beneath eight states and is heavily used.
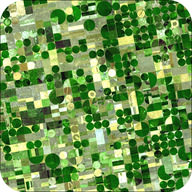
Figure 14. Farms in Kansas
The water in the aquifer is mostly from the last ice age. About eight times more water is taken from the Ogallala Aquifer each year than is replenished. Much of the water is used for irrigation .Farms in Kansas use central pivot irrigation (Figure 14), which is more efficient since water falls directly on the crops instead of being shot in the air. These fields are between 800 and 1600 meters (0.5 and 1 mile) in diameter.
Subsidence
Lowering the water table may cause the ground surface to sink. Subsidence may occur beneath houses and other structures. The San Joaquin Valley of California is one of the world’s major agricultural areas. So much groundwater has been pumped that the land has subsided many tens of feet.
Salt Water Intrusion
When coastal aquifers are overused, salt water from the ocean may enter the aquifer, contaminating the aquifer and making it less useful for drinking and irrigation. Salt water incursion is a problem in developed coastal regions, such as on Hawaii.
Summary
- When water is pumped from an aquifer, the water table declines and wells must be drilled deeper.
- The Ogallala Aquifer was filled in the ice age but is being used to irrigate the farms of the Midwestern U.S. at a rate far greater than it is being replenished.
- Ground subsidence and saltwater intrusion are two possible consequences of groundwater overuse.
Practice
Use this resource to answer the questions that follow.
- How has irrigation changed farming?
- What is leading to people’s demands for additional water?
- What do scientists need to see to better plan for future water use?
- What is the GRACE satellite doing?
- How does GRACE find groundwater aquifers?
- How people know the aquifers are being depleted?
- What is happening in India? what will happen if the water continues to decline?
- What is the future of water?
Quality of Groundwater
As was noted at the very beginning of this chapter, one of the good things about groundwater as a source of water is that it is not as easily contaminated as surface water is. But there are two caveats to that: one is that groundwater can become naturally contaminated because of its very close connection to the materials of its aquifer, and the second is that once contaminated by human activities, groundwater is very difficult to clean up.
Natural Contamination of Groundwater
Groundwater moves slowly through an aquifer, and unlike the surface water of a stream, it has a lot of contact with the surrounding rock or sediment. In most aquifers, the geological materials that make up the aquifer are relatively inert, or are made up of minerals that dissolve very slowly into the groundwater. Over time, however, all groundwater gradually has more and more material dissolved within it as it remains in contact with the aquifer. In some areas, that rock or sediment includes some minerals that could potentially contaminate the water with elements that might make the water less than ideal for human consumption or agricultural use. Examples include copper, arsenic, mercury, fluorine, sodium, and boron. In some cases, contamination may occur because the aquifer material has particularly high levels of the element in question. In other cases, the aquifer material is just normal rock or sediment, but some particular feature of the water or the aquifer allows the contaminant to build up to significant levels.
An example of natural contamination takes place in the bedrock aquifers of the east coast of Vancouver Island and the adjacent Gulf Islands. The aquifer is the Cretaceous (90 Ma to 65 Ma) Nanaimo Group, which is made up of sandstone, mudstone, and conglomerate (Figure 15).
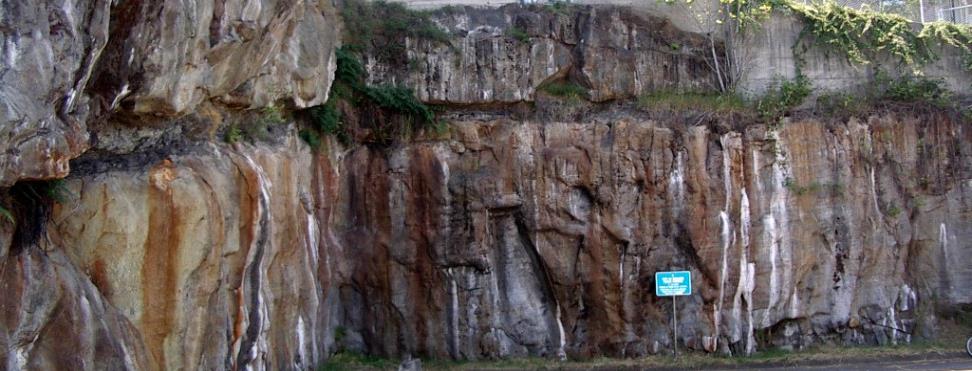
Figure 15. Cretaceous Nanaimo Group sandstone exposed in a Nanaimo parking lot [SE]
The rocks of the Nanaimo Group are not particularly enriched in any trace elements, but the submarine-fan sandstone that makes up much of the group is a lithic wacke, and therefore has relatively high levels of clay (for a sandstone). This clay is good at adsorbing[1] some elements from the water and desorbing others, and in the process, its pH goes up (it becomes alkaline). At high pH levels (some as high as 9 in the Nanaimo Group), the element fluorine that is present naturally in the rock (as it is in almost any rock) has an increased tendency to dissolve in the water. In some areas, groundwater in the Nanaimo Group has fluorine levels that are well above recommended levels for drinking water. The World Health Organization (WHO) maximum acceptable concentration (MAC) for fluorine is 1.5 mg/L (milligrams per litre). Between 5% and 10% of the domestic wells around Nanaimo and adjacent Gabriola Island have more than that, some as much as 10 mg/L. A small amount of fluorine in the human diet is considered important for maintaining dental health, but high levels can lead to malformation and discolouration of teeth, and long-term exposure can lead to other more serious health effects such as skeletal problems.
Nanaimo Group groundwater can also have elevated levels of boron, again related to pH and adsorption from clay minerals. While boron at the levels found there is not toxic to humans, there is enough boron in some wells to be toxic to plants, and the water cannot be used for irrigation.
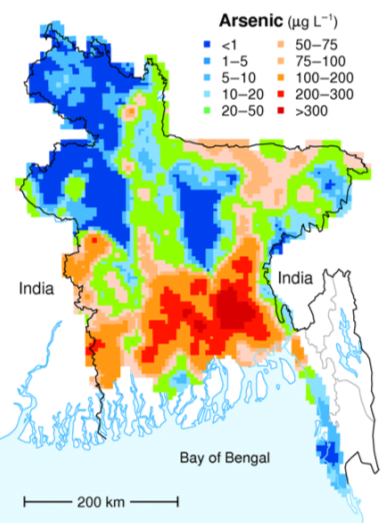
Figure 16. The distribution of arsenic in groundwater in Bangladesh. The WHO recommended safe level for arsenic is 10 μg/L. All of the green, orange, and red areas on the map exceed that limit. [From: BGS and DPHE. 2001. Arsenic contamination of groundwater in Bangladesh. Kinniburgh, D G and Smedley, P L, http://www.bgs.ac.uk/arsenic/bangladesh/.]
Rural residents in the densely populated country of Bangladesh (over 1,000 residents/km2, compared with 3.4/km2 in Canada) used to rely mostly on surface supplies for their drinking water, and many of these were subject to bacterial contamination. Infant mortality rates were among the highest in the world and other illnesses such as diarrhea, dysentery, typhoid, cholera, and hepatitis were common. In the 1970s, international agencies, including UNICEF, started a program of drilling wells to access abundant groundwater supplies at depths of 20 m to 100 m. Eventually over 8 million such wells were drilled. Infant mortality and illness rates dropped dramatically, but it was later discovered that the water from a high proportion of these wells has arsenic above safe levels (Figure 16).
Most of the wells in the affected areas are drilled into relatively recent sediments of the vast delta of the Ganges and Brahmaputra Rivers. While these sediments are not particularly enriched in arsenic, they have enough organic matter in them to use up any oxygen present. This leads to water with a naturally low oxidation potential (anoxic conditions); arsenic is highly soluble under these conditions, and so any arsenic present in the sediments easily gets dissolved into the groundwater. Arsenic poisoning leads to headaches, confusion, and diarrhea, and eventually to vomiting, stomach pain, and convulsions. If not treated, the final outcomes are heart disease, stroke, cancer, diabetes, coma, and death. There are ways to treat arsenic-rich groundwater, but it is a challenge in Bangladesh to implement the simple and effective technology that is available.
Anthropogenic Contamination of Groundwater
Groundwater can become contaminated by pollution at the surface (or at depth), and there are many different anthropogenic (human-caused) sources of contamination.
The vulnerability of aquifers to pollution depends on several factors, including the depth to the water table, the permeability of the material between the surface and the aquifer, the permeability of the aquifer, the slope of the surface, and the amount of precipitation. Confined aquifers tend to be much less vulnerable than unconfined ones, and deeper aquifers are less vulnerable than shallow ones. Steeper slopes mean that surface water tends to run off rather than infiltrate (and this can reduce the possibility of contamination). Contamination risk is also less in dry areas than in areas with heavy rainfall.
Studies of groundwater vulnerability have been completed for various regions of British Columbia. A groundwater vulnerability map for southern Vancouver Island is shown in Figure 17. The yellow to red areas are considered to have high vulnerability to pollution from surface sources, and most of these are where the aquifers are unconfined in quite permeable unconsolidated sediments of either glacial or fluvial origin, where the water table is relatively shallow and the terrain is relatively flat.
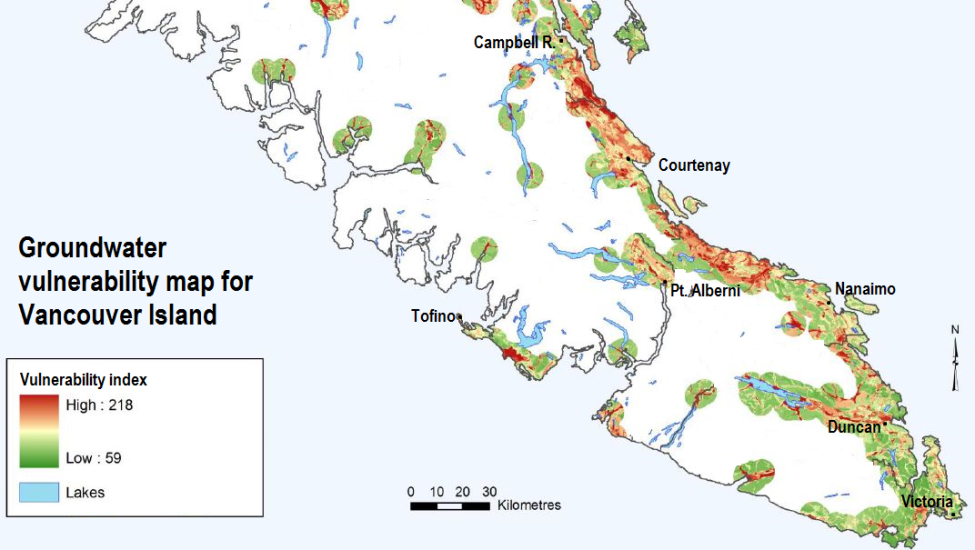
Figure 17. The vulnerability to anthropogenic contamination of aquifers on southern Vancouver Island. Much of the island is not mapped (shown as white) because of a lack of aquifer information in areas without wells. [From: Newton, P. and Gilchrist, A. 2010. Technical summary of intrinsic vulnerability mapping methods of Vancouver Island, Vancouver Island Water Resources Vulnerability Mapping Project, Vancouver Island University, 45pp. Used with permission. https://web.viu.ca/groundwater/PDF/VI_DRASTIC_Summary_Phase2_2010.pdf]
The important sources of anthropogenic groundwater contamination include the following:
- Chemicals and animal waste related to agriculture, and chemicals applied to golf courses and domestic gardens
- Landfills
- Industrial operations
- Mines, quarries, and other rock excavations
- Leaking fuel storage tanks (especially those at gas stations)
- Septic systems
- Runoff from roads (e.g., winter salting) or chemical spills of materials being transported
Agriculture
Intensive agricultural operations and golf courses can have a significant impact on the environment, especially where chemicals and other materials are used to enhance growth or control pests. An example of agricultural contamination is in the Abbotsford area of the Fraser Valley, where nitrate levels above the 44 mg/L maximum acceptable level (expressed as nitrate) in the Abbotsford-Sumas aquifer have been observed since the 1950s; however, the problem became much worse as agriculture intensity increased in the 1980s. By 2004, groundwater with nitrate levels in excess of 44 mg/L was reported over an area of about 75 km2 around Abbotsford, and the problem extended across the border into the Sumas area of Washington State.
This region is intensively used for berry crops (especially raspberries and blueberries) and large poultry operations, as well as lesser amounts of grazing and forage crops. Chicken manure is typically stored in fields adjacent to chicken barns, and may release nitrogen to the environment from runoff water, and from releases of ammonia gas. Over decades, both chemical fertilizers and chicken manure and other manures have been applied to the berry crops to provide extra nitrogen to help maximize berry growth. If the fertilizer added is in excess of what the plants need, or is poorly timed compared to when it is needed, then the extra nitrogen may be leached into the groundwater below. Berry crops are irrigated over the summer to help the crops grow. Summer irrigation and winter rainfall may carry excess nitrate from the near surface to the aquifer below.
Since the 1990s, agricultural practices have been tightened up to reduce the rate of groundwater contamination, but it will take decades for nitrate levels to drop in the Abbotsford-Sumas aquifer. Agriculture and Agri-Food Canada and many others are conducting research on better irrigation and nitrate management techniques to reduce the amount of nitrogen that leaches to groundwater.
Landfills
In the past, domestic and commercial refuse was commonly trucked to a “dump” (typically a hole in the ground), and when the hole was filled, it was covered with soil and forgotten. In situations like this, rain and melting snow can easily pass through the soil used to cover the refuse. This water passes into the waste itself, and the resulting landfill leachate that flows from the bottom of the landfill can seriously contaminate the surrounding groundwater and surface water. In the past few decades, regulations around refuse disposal have been significantly strengthened, and important steps have been taken to reduce the amount of landfill waste by diverting recyclable and compostable materials to other locations.
A modern engineered landfill has an impermeable liner (typically heavy plastic, although engineered clay liners or natural clay may be adequate in some cases), a plumbing system for draining leachate (the rainwater that flows through the refuse and becomes contaminated), and a network of monitoring wells both within and around the landfill (Figure 18). Once part or all of a landfill site is full, it is sealed over with a plastic cover, and a system is put in place to extract landfill gas (typically a mixture of carbon dioxide and methane). That gas can be sent to a nearby location where it is burned to create heat or used to generate electricity. The leachate must be treated, and that can be done in a normal sewage treatment plant.
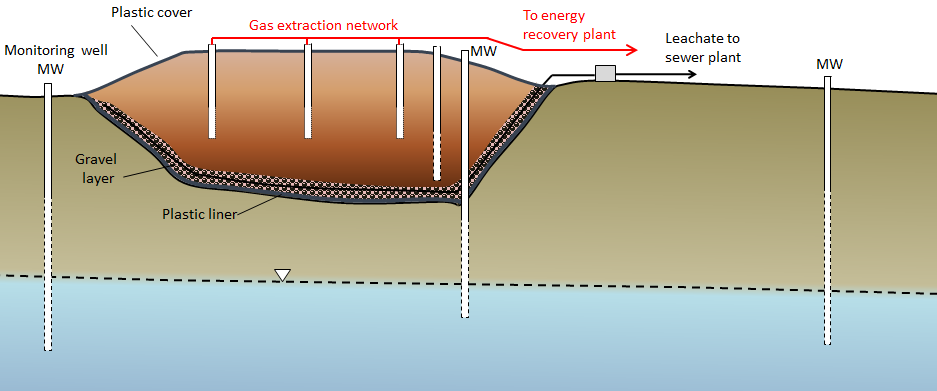
Figure 18. A cross-section of a typical modern landfill [SE]
The monitoring wells are used to assess the level of the water table around the landfill and to collect groundwater samples so that any leakage can be detected. Because some leakage is almost inevitable, the ideal placement for landfills is in areas where the depth to the water table is significant (tens of metres if possible) and where the aquifer material is relatively impermeable. Landfills should also be situated far from streams, lakes, or wetlands so that contamination of aquatic habitats can be avoided.
Today there are hundreds of abandoned dumps scattered across the country; most have been left to contaminate groundwater that we might wish to use sometime in the future. In many cases, it’s unlikely that we’ll be able to do so.
Exercises: What Goes on at Your Landfill?
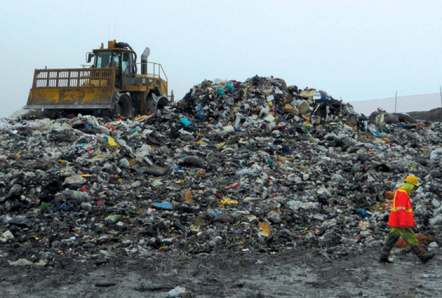
Figure 19. A landfill [SE photo]
Unless you live in a remote rural area, there’s a good chance that the refuse you can’t recycle is picked up at the curb and taken to a landfill. Most landfills are operated by cities or regional districts, and you should be able to find information about yours on the appropriate local government website. See if you can answer some the following questions:
- Which government body operates your landfill?
- Where is the landfill situated?
- Is your waste all placed in a landfill, or are there other processes in use (e.g., incineration or composting)?
- Are landfill gases captured, and, if so, what is done with them?
- What could be changed to improve the waste disposal situation in your community (e.g., more recycling, compost collection, waste-to-energy technology)?
Industrial Operations
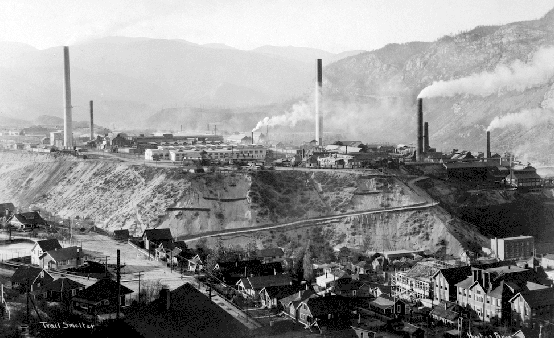
Figure 20. The Trail lead-zinc smelter in 1929
[http://upload.wikimedia.org /wikipedia/commons/2/20 /Trail_Smelter_in_Year_1929.png]
Although western Canada doesn’t have the same extent of industrial pollution as other parts of the country, there are still seriously contaminated sites in the west, most with the potential to contaminate groundwater. One example is the lead and zinc smelter at Trail, B.C. The largest in the world, it has been operating for over 100 years and has left a residue of metal contamination around the region (Figure 20). In some parts of Trail, the contamination is serious enough that existing soil has been removed from residential properties and replaced with clean soil brought in from elsewhere. This contaminated soil has contributed to contamination of groundwater in the Trail area. Groundwater beneath the actual smelter site is contaminated, and the operator (Teck Resources) is currently working on plans to prevent that water from reaching the nearby Columbia River.
Mines, Quarries, and Rock Excavations
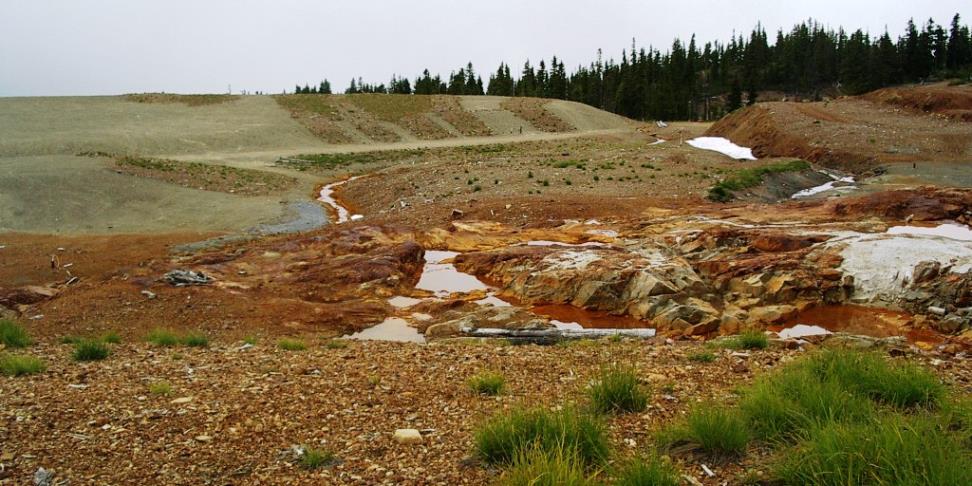
Figure 21. Acidic runoff at the abandoned Mt. Washington Mine near Courtenay, B.C. [SE]
Mines and other operations that involve the excavation of large amounts of rock (e.g., highway construction) have the potential to create serious environmental damage. The exposure of rock that has previously not been exposed to air and water can lead to the oxidation of sulphide-bearing minerals, such a pyrite (FeS2), within the rock. The combination of pyrite, water, oxygen, and a special type of bacteria (Acidithiobacillus ferrooxidans) that thrives in acidic conditions leads to the generation of acidity, in some cases to pH less than 2. Water that acidic is hazardous by itself, but the low pH also has the property of increasing the solubility of certain heavy metals. The water that is generated by this process is known as acid rock drainage (ARD). ARD can occur naturally where sulphide-bearing rocks are near the surface. The issue of ARD is a major environmental concern at both operating mines and abandoned mines. In streams around the Mt. Washington Mine on Vancouver Island (Figure 21), copper levels are high enough to be toxic to fish. Groundwater adjacent to the contaminated streams in the area is very likely contaminated as well.
Leaking Fuel Tanks
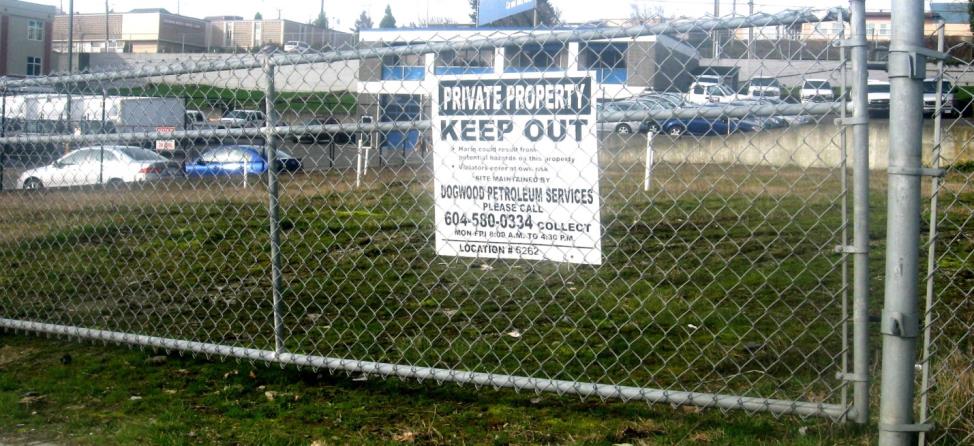
Figure 22. A closed and fenced gas station site in Nanaimo, B.C. The white pipes in the background are wells for monitoring groundwater contamination on the site. [SE]
Underground storage tanks (USTs) are used to store fuel at gas stations, industrial sites, airports, and anywhere that large volumes of fuel are used. They do not last forever, and eventually they start to leak their contents into the ground. This is a particular problem at older gas stations — although it may also become a future problem at newer gas stations. You may have noticed gas stations that have been closed and then surrounded by chain-link fence (Figure 22). In virtually all such cases the closure has been triggered by the discovery of leaking USTs and the requirement to cease operations and remediate the site.Petroleum fuels are complex mixtures of hydrocarbon compounds and the properties of their components—such as density, viscosity, solubility in water, and volatility — tend to vary widely. As a result, a petroleum spill is like several spills for the price of one. The petroleum liquid slowly settles through the unsaturated zone and then tends to float on the surface of the groundwater (Figure 23). The more readily soluble components of the spill dissolve in the groundwater and are dispersed along with the normal groundwater flow, and the more volatile components of the spill rise toward the surface, potentially contaminating buildings.
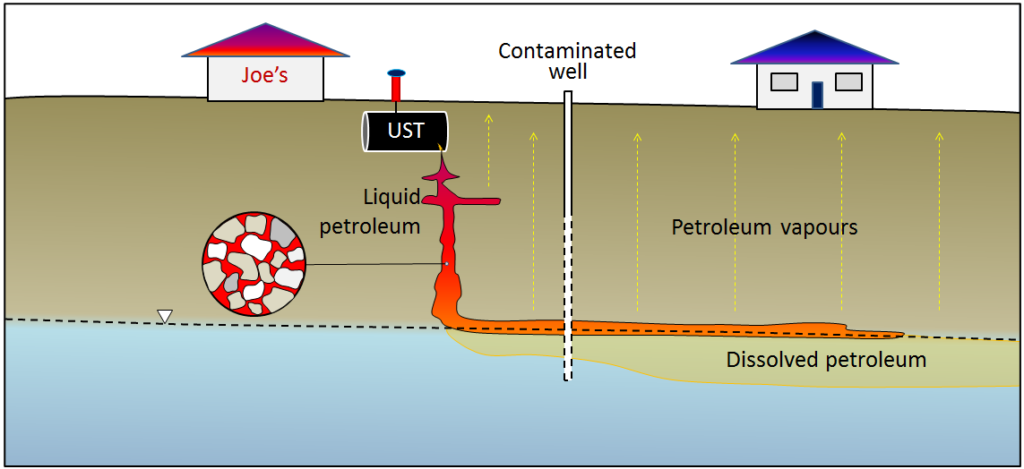
Figure 23. A depiction of the fate of different components of a petroleum spill from an underground storage tank. [SE]
Exercises: Find a Leaking UST in Your Community
There is almost certainly a leaking UST at a former gas station near you. Look for an empty property that is surrounded by a chain-link fence with “No Trespassing” signs. You might see evidence of monitoring wells (like those shown in Figure 23), and there could be some petroleum barrels around that are being used to store contaminated water. Once you’ve identified one of these, you’ll probably start seeing them everywhere!
Septic Systems
In areas that are not served by sewage networks leading to a central sewage treatment plant, most homeowners rely on septic systems for disposal of sewage. There are two primary components to a simple septic system, the septic tank and the drainage field (Figure 24). A typical septic tank is constructed of either concrete or plastic and has a volume of 5,000 L to 10,000 L (5 m3 to 10 m3). This forms the first treatment and is designed to be anaerobic (without oxygen). That promotes the activity of certain bacteria that help break down the waste. As the waste is degraded, some portions tend to sink to form sludge at the base of the tank, and others float to the surface, forming a scum layer. A septic tank may be divided into two parts to keep the sludge at the bottom and the scum on the top from draining out. The water then moves to the drainage field, which provides the right conditions for a different set of bacteria that operate in aerobic conditions. The drainage field includes an array of plastic pipes that are perforated to allow the effluent to drain out over a large area and seep slowly into the ground. In order to install a drainage field, it is first necessary to test the soil below, as it must be sufficiently permeable to allow the effluent to percolate away, but not so permeable that it flows too quickly and the soil is not able to filter out the pathogenic bacteria.
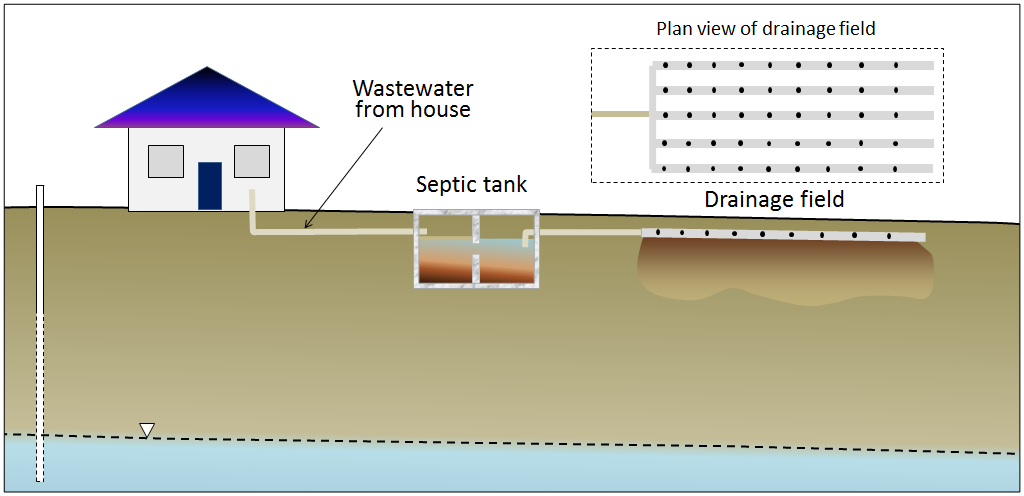
Figure 24. A typical septic system. [SE]
If they are properly installed and used, and if the sludge is periodically removed from the tank, a septic system should be effective in treating the sewage for decades. The anaerobic and aerobic bacteria should be able to break down the incoming waste and there should be little risk to the surface environment or groundwater. But many things can go wrong with a septic system, including the following:
- If inappropriate chemicals are added to the waste stream, they may interfere with the natural breakdown of the sewage.
- If the tank is not periodically pumped out, solids can get into the drainage field and compromise the drainage, resulting in the flow of effluent toward the surface.
- If the soil is either not sufficiently permeable or too permeable, the effluent will not drain away (and will start to pool at the surface) or it will drain too quickly.
- If the drainage field is constructed in an area where the water table is close to surface, some of the effluent is likely to flow into the groundwater without being treated.
Prevention and Mitigation of Groundwater Contamination
As illustrated in the landfill example above, there are two fairly simple ways to significantly reduce the chance and degree of groundwater contamination from surface sources. One is to prevent rainwater from infiltrating down to the water table and picking up contaminants; this can be achieved by simply capping or roofing over the landfill, mine tailings, or spill site. The second is to provide an impermeable barrier beneath the contaminant. Modern landfills and mine tailings impoundments are all built using some combination of clay and engineered plastic barriers. Both of these solutions — caps and liners — are subject to failure due to leaks.
Once contaminants are in the groundwater, the main form of remediation is to pump out the contaminated water and treat it at the surface. This can be a slow process, and preventing the contaminant from travelling significantly during this process can be accomplished by manipulating local groundwater flow through the extraction or injection of water at certain locations. Consider this in the exercise below.
Exercises: Manipulating a Contaminant Plume
This diagram shows a groundwater contaminant plume in red. The source of the contamination has been removed but if the plume is not dealt with, it will eventually enter the stream and threaten the health of wildlife. Pumping the contaminant from well B for treatment will not be sufficient to prevent some of the contamination from making it to the stream.
What could you do at wells A and C to prevent this? Explain and use the diagram below to illustrate the expected changes to the water table and the movement of the plume.
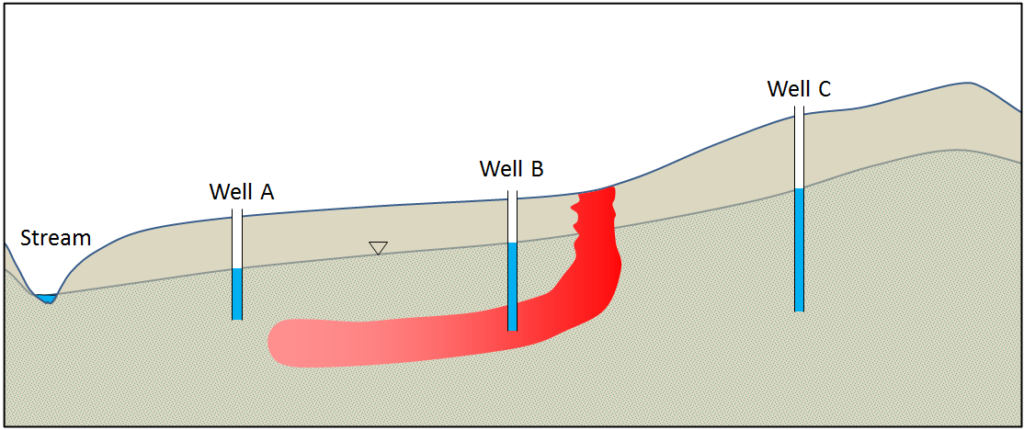
Figure 25. Contaminant Plume
Check Your Understanding
Answer the question(s) below to see how well you understand the topics covered in the previous section. This short quiz does not count toward your grade in the class, and you can retake it an unlimited number of times.
Use this quiz to check your understanding and decide whether to (1) study the previous section further or (2) move on to the next section.
Check Your Understanding
The water table is located __________.
- at the surface.
- at the top of the saturated zone.
- at the bottom of the saturated zone.
Show Answer
at the top of the saturated zone.
Candela Citations
- Introduction to Components of Groundwater. Authored by: Kimberly Schulte and Lumen Learning. Provided by: Lumen Learning. License: CC BY: Attribution
- 13.3: Groundwater. Provided by: CK-12. Located at: http://www.ck12.org/book/CK-12-Earth-Science-For-High-School/section/13.3/. License: CC BY-NC: Attribution-NonCommercial
- Back to Basics on Groundwater. Authored by: Matt Herod. Provided by: European Geosciences Union. Located at: http://blogs.egu.eu/network/geosphere/2013/09/17/back-to-basics-on-groundwater/. License: CC BY: Attribution
- Groundwater Depletion. Provided by: CK-12. Located at: http://www.ck12.org/earth-science/Groundwater-Depletion/lesson/Groundwater-Depletion-HS-ES/. License: CC BY-NC: Attribution-NonCommercial
- Groundwater Quality. Authored by: Steven Earle. Provided by: BC Campus. Located at: https://opentextbc.ca/geology/chapter/14-4-groundwater-quality. Project: Physical Geology. License: CC BY: Attribution
- NASA | Science for a Hungry World: Part 5. Authored by: NASA Goddard. Located at: https://youtu.be/o1QsCa7RmmU. License: All Rights Reserved. License Terms: Standard YouTube License
- Aquifers. Provided by: USGS. Located at: http://water.usgs.gov/edu/earthgwaquifer.html. License: Public Domain: No Known Copyright
- Groundwater: Wells. Authored by: Roger M. Waller. Provided by: USGS. Located at: http://water.usgs.gov/edu/earthgwwells.html. Project: Ground Water and the Rural Homeowner. License: Public Domain: No Known Copyright
- “Adsorb” (with a “d”) is not the same as “absorb” (with a “b”). Water can be absorbed by a sponge. Ions dissolved in water can be adsorbed onto—or desorbed from—the surfaces of clay minerals. ↵