Critique and interpret major types of evidence supporting the Theory of Plate Tectonics.
Plate tectonics is the most important concept in modern geology. This section will introduce you to the concept of plate tectonics, its various processes and mechanisms, and how it shapes our world.
What You’ll Learn to Do
- Describe and compare different types of plate motions, rates of motion and the driving mechanisms and forces involved with each.
- Evaluate various types of plate boundaries.
- Recognize how plate tectonics–An exemplary Theory– links seemingly different geologic features, and makes testable predictions!
Theory of Plate Tectonics
When the concept of seafloor spreading came along, scientists recognized that it was the mechanism to explain how continents could move around Earth’s surface. Like the scientists before us, we will now merge the ideas of continental drift and seafloor spreading into the theory of plate tectonics.
Earth’s Tectonic Plates
Seafloor and continents move around on Earth’s surface, but what is actually moving? Really, it’s more about the sea floor BETWEEN the continents that simply comes (spreading ridges) and goes (subduction zones)
A lithospheric plate is the outer rigid part of the earth– it consists of the upper mantle and the crust, either continental or oceanic.
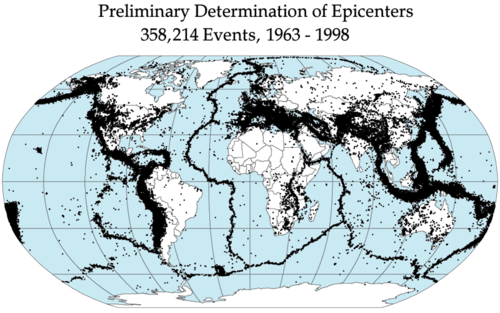
Figure 1. Earthquakes outline the plates.
During the 1950s and early 1960s, scientists set up seismograph networks to see if enemy nations were testing atomic bombs. These seismographs also recorded all of the earthquakes around the planet. The seismic records could be used to locate an earthquake’s epicenter, the point on Earth’s surface directly above the place where the earthquake occurs.
Earthquake epicenters outline the plates. Mid-ocean ridges, trenches, and large faults mark the edges of the plates, and this is where earthquakes occur (figure 1).
In general, a lithospheric plate is a big thing!
Lithospheric plates are NOT just some slabs of rock; instead they are major portions of continents and oceans.
The lithosphere is divided into a dozen major and several minor plates (figure 2). The plates’ edges can be drawn by connecting the dots that mark earthquakes’ epicenters. A single plate can be made of all oceanic lithosphere or all continental lithosphere, but most plates are made of a combination of both. Just take a look at the African Plate– it’s not just the continent of Africa, but also a large amount of adjoining oceanic lithosphere.
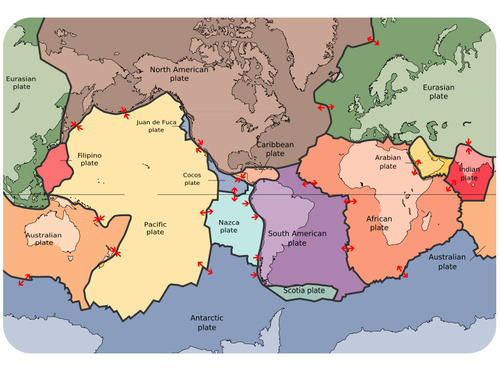
Figure 2. The lithospheric plates and their names. The arrows show whether the plates are moving apart, moving together, or sliding past each other.
Movement of the plates over Earth’s surface is termed plate tectonics. Plates move at a rate of a few centimeters a year, about the same rate fingernails grow.
How Plates Move
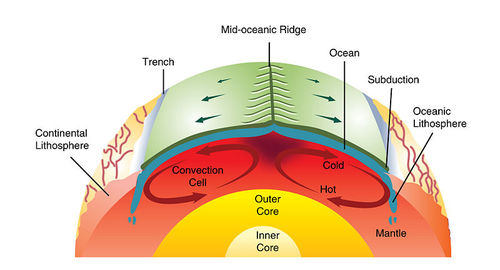
Figure 3. Mantle convection drives plate tectonics. Hot material rises at mid-ocean ridges and sinks at deep sea trenches, which keeps the plates moving along the Earth’s surface.
So, if the appearance (at mid ocean ridges) of new ocean floor and the disappearance (at subduction zones) of older ocean floor is the key, how does it work??
Answer:
Picture two convection cells side-by-side in the mantle, similar to the illustration in figure 3.
- Hot “plastic” asthenospheric mantle from the two adjacent cells rises at the ridge axis– it’s not liquid remember, but instead more like silly putty or taffy-like material.
- Just below the ridge axis, the rising plastic mantle undergoes melting, becoming an actual liquid– basalt magma. As it cools, it creates ocean crust.
- At the outer limbs of these convection cells, the oceanic lithosphere (crust and upper mantle) plunge down into the deeper mantle. This takes place at the deep sea trenches.The ultimate fate of a subducted slab of oceanic lithosphere is not that clear– it probably is eventually absorbed into the deeper mantle below.
Check out this animation of mantle convection and watch this video:
https://youtube.com/watch?v=p0dWF_3PYh4%3Fenablejsapi%3D1
Driving Forces for Plate Motion
The primary reason that the earth, unlike other planets, has active plate motion (i.e. a rigid outer shell of the planet, broken into chunks, that move around with time!)–
Is that the earth has a hot interior and has a plastic, taffy-like, region called the asthenosphere that is mechanically de-coupled from the overlying rigid layer called the lithosphere.
“De-coupling” means that the more rigid lithosphere can slide over the asthenosphere.
But WHY does it move?!
Geophysicists have identified three big factors:
- Convective drag. As the underlying asthenosphere moves it can generate a frictional drag on the overlying lithosphere. Remember, the asthenosphere is NOT a liquid, but it moves like toothpaste, or taffy, or warm-butter, or something like that! At any rate, this is not considered to be a particularly significant driver of plate motion in most locations.
- Slab-Pull. As a subducted plate descends into the mantle (see “convergent boundaries” below), the thick slab of primarily basaltic material (perhaps 100km, 40-60miles, thick!) undergoes mineralogic changes such that the slab becomes denser. An example of this is the conversion of pyroxene to garnet. The key is that this increased density serves to pull the slab even more, taking it down to great depth. (What happens to lithospheric slabs that descend into the mantle?– we don’t really know, but they are probably resorbed in some fashion, adding amongst other things water to the mantle.)
- Ridge-Push. This is probably one of the more “forceful” of the driving motions for plate tectonics, because it’s linked to gravity! The mid-ocean ridges are high areas (perhaps a mere 1-2km beneath the waves above; whereas the deep abyssal plains, which make up the bulk of the big ocean basins, are around 5km in depth). The high ridge regions, because their lithosphere can slide, simply do just that and slide downwards off the ridge crest!
Plate Boundaries
Plate boundaries are literally the places where two different plates are in contact. These are generally places with some geologic “action”– things like volcanoes, earthquakes, and mountain building.
What sort of plate boundaries exist? In reality, there are only three primary types!
- Divergent plate boundaries: the two plates move away from each other.
- Convergent plate boundaries: the two plates move towards each other.
- Transform plate boundaries: the two plates slip past each other.
The type of plate boundary and the type of crust found on each side of the boundary is an important predictor of what sort of rocks and deformation are expected.
For example,
In general, we find earthquakes of all magnitude (small to big) at convergent and transform boundaries, with the largest quakes (e.g. magnitude 8-9) being limited to convergent boundaries. Earthquakes of magnitude greater than Richter-Mag 9 (or so) just don’t occur, because rocks cannot build up enough stress to release more energy. In contrast, divergent boundaries typically have earthquakes of lower magnitude– occasionally Richter-Mag 6, but typically less than 5.
Divergent Plate Boundaries
Plates move apart at mid-ocean ridges where new seafloor forms. Between the two plates is a rift valley. Lava flows at the surface cool rapidly to become basalt, but deeper in the crust, magma cools more slowly to form gabbro. So the entire ridge system is made up of igneous rock that is either extrusive or intrusive. Earthquakes are common at mid-ocean ridges since the movement of magma and oceanic crust results in crustal shaking, but as mentioned above the earthquakes are generally rather weak.
Iceland is an unusual place– a mid-ocean ridge that breaches the ocean surface and is above sea-level. The vast majority of mid-ocean ridges are located deep below the sea (figure 4).
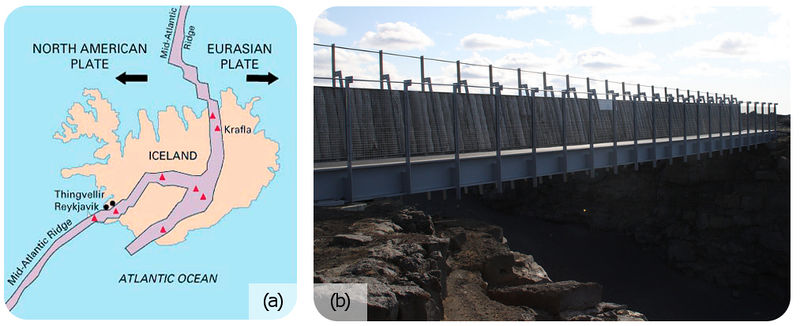
Figure 4. (a) Iceland is the one location where the ridge is located on land: the Mid-Atlantic Ridge separates the North American and Eurasian plates; (b) The rift valley in the Mid-Atlantic Ridge on Iceland.
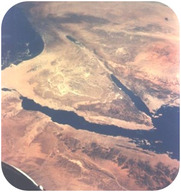
Figure 5. The Arabian, Indian, and African plates are rifting apart. The Red Sea (very edge on the lower right) is a rift zone, as are the Gulf of Aqaba and the Gulf of Suez– the two prongs or ears in the image above!
Check out these animations:
Can divergent plate boundaries occur within a continent? What is the result? In continental rifting (figure 5), magma rises beneath the continent, causing it to become thinner, break, and ultimately split apart. New ocean crust erupts in the void, creating an ocean between continents.
Convergent Plate Boundaries
When two plates converge, the result depends on the type of lithosphere the plates are made of. No matter what, smashing two enormous slabs of lithosphere together results in magma generation and earthquakes.
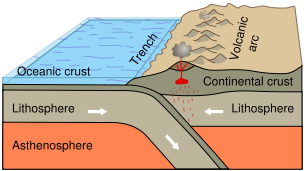
Figure 6. Subduction of an oceanic plate beneath a continental plate causes earthquakes and forms a line of volcanoes known as a continental arc.
Ocean-Continent
When oceanic crust converges with continental crust, the denser oceanic plate plunges beneath the continental plate. This process, called subduction, creates oceanic trenches (figure 6) and magmatic activity above the subducted slab.
The entire region is known as a subduction zone. Subduction zones are marked by earthquakes (associated with slip on the down-going slab) and volcanic eruptions.
The subducting plate causes melting in the mantle lithosphere that lies directly above the subducting slab. The magma rises and erupts, creating volcanoes. The chain of volcanos above a subducting plate (figure 7) is a volcanic arc, in this case a continental arc (as opposed to an oceanic volcanic arc).
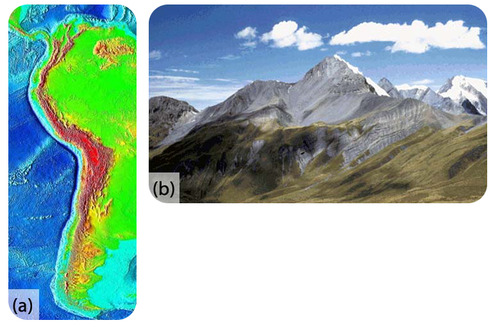
Figure 7. (a) At the trench lining the western margin of South America, the Nazca plate is subducting beneath the South American plate, resulting in the Andes Mountains (brown and red uplands); (b) Convergence has pushed up limestone in the Andes Mountains where volcanoes are common.
Take a look at this map of earthquake epicenters at subduction zones.
And here, This animation shows the relationship between subduction of the lithosphere and creation of a volcanic arc.
The volcanoes of northeastern California—Lassen Peak, Mount Shasta, and Medicine Lake volcano—along with the rest of the Cascade Mountains of the Pacific Northwest are the result of subduction of the Juan de Fuca plate beneath the North American plate (figure 8). The Juan de Fuca plate is created by seafloor spreading just offshore at the Juan de Fuca ridge.
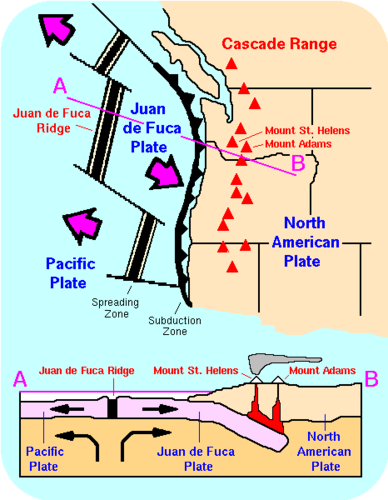
Figure 8. The Cascade Mountains of the Pacific Northwest are a continental arc.
If the magma at a continental arc is felsic, it may be too viscous (thick) to rise through the crust. The magma will cool slowly to form granite or granodiorite. These large bodies of intrusive igneous rocks are called batholiths, which may someday be uplifted to form a mountain range (figure 9).
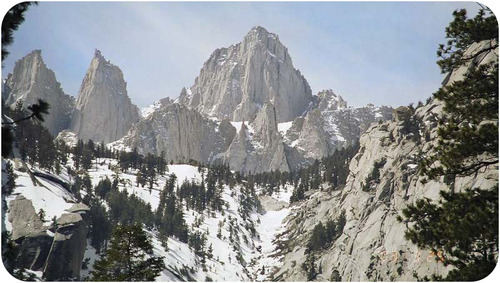
Figure 9. The Sierra Nevada batholith cooled beneath a volcanic arc roughly 200 million years ago. The rock is well exposed here at Mount Whitney. Similar batholiths are likely forming beneath the Andes and Cascades today. In other words— the rock of these mountains is the now cooled deep magma chambers that were located above a subducting slab, but beneath overlying volcanoes!
Ocean-Ocean
When two oceanic plates converge, the older, denser plate will subduct into the mantle. An ocean trench marks the location where the plate is pushed down into the mantle. The line of volcanoes that grows on the upper oceanic plate is an island arc, or oceanic arc. Do you think earthquakes are common in these regions (figure 10)?
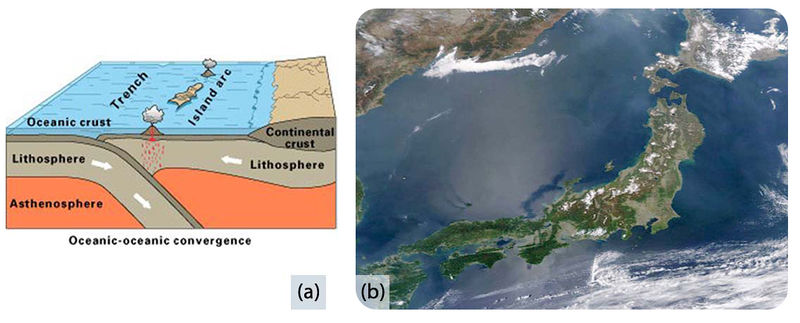
Figure 10. (a) Subduction of an ocean plate beneath an ocean plate results in a volcanic island arc, an ocean trench and many earthquakes. (b) Japan is an arc-shaped island arc composed of volcanoes off the Asian mainland, as seen in this satellite image.
Check out this animation of an ocean continent plate boundary.
Continent-Continent
Continental plates are too buoyant to subduct. What happens to continental material when it collides? Since it has nowhere to go but up, this creates some of the world’s largest mountains ranges (figure 11). Magma cannot penetrate this thick crust so there are no volcanoes, although the magma stays in the crust. Metamorphic rocks are common because of the stress the continental crust experiences. With enormous slabs of crust smashing together, continent-continent collisions bring on numerous and large earthquakes.
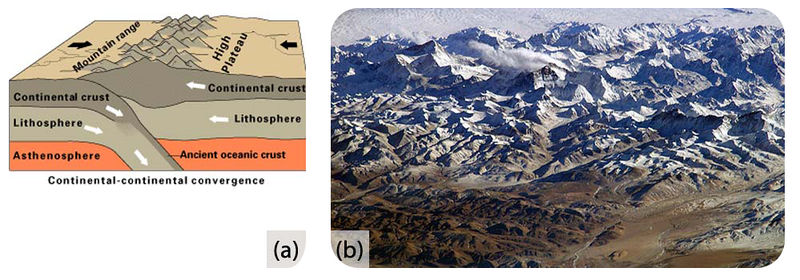
Figure 11. (a) In continent-continent convergence, the plates push upward to create a high mountain range. (b) The world’s highest mountains, the Himalayas, are the result of the collision of the Indian Plate with the Eurasian Plate, seen in this photo from the International Space Station.
Check out this short animation of the Indian Plate colliding with the Eurasian Plate.
Watch this animation of the Himalaya rising.
The Appalachian Mountains are the remnants of a large mountain range that was created when North America rammed into Eurasia about 250 million years ago.
Transform Plate Boundaries
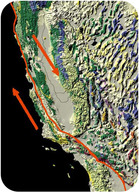
Figure 12. At the San Andreas Fault in California, the Pacific Plate is sliding northwest relative to the North American plate, which is moving southeast. At the northern end of the picture, the transform boundary turns into a subduction zone.
Transform plate boundaries are seen as transform faults, where two plates move past each other in opposite directions. Transform faults on continents bring massive earthquakes (figure 12).
California is very geologically active. What are the three major plate boundaries in or near California (figure 13)?
- A transform plate boundary between the Pacific and North American plates creates the San Andreas Fault, the world’s most notorious transform fault.
- Just offshore, a divergent plate boundary, Juan de Fuca ridge, creates the Juan de Fuca plate.
- A convergent plate boundary between the Juan de Fuca oceanic plate and the North American continental plate creates the Cascades volcanoes.
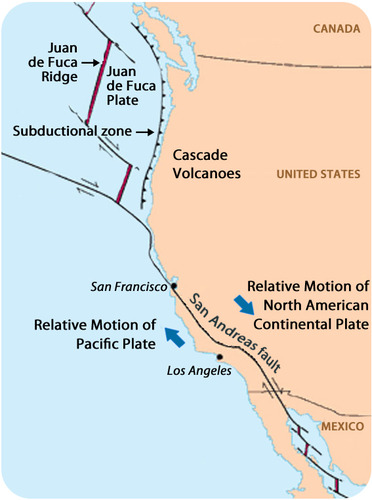
Figure 13. This map shows the three major plate boundaries in or near California.
A brief review of the three types of plate boundaries and the structures that are found there is the subject of this wordless video.
Earth’s Changing Surface
Geologists know that Wegener was right because the movements of continents explain so much about the geology we see. Most of the geologic activity that we see on the planet today is because of the interactions of the moving plates.
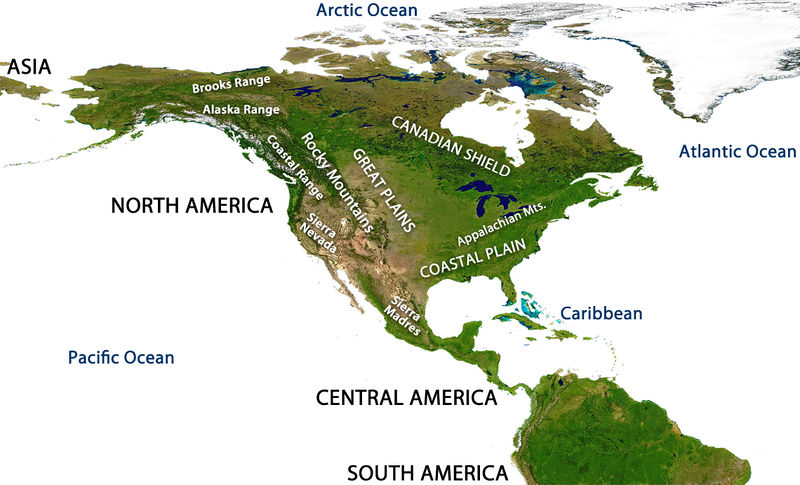
Figure 14. Mountain ranges of North America.
In the map of North America (figure 14), where are the mountain ranges located? Using what you have learned about plate tectonics, try to answer the following questions:
- What is the geologic origin of the Cascades Range? The Cascades are a chain of volcanoes in the Pacific Northwest. They are not labelled on the diagram but they lie between the Sierra Nevada and the Coastal Range.
- What is the geologic origin of the Sierra Nevada? (Hint: These mountains are made of granitic intrusions.)
- What is the geologic origin of the Appalachian Mountains along the Eastern US?
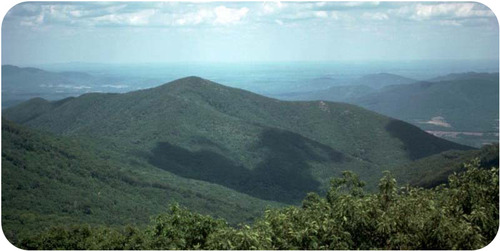
Figure 15. About 200 million years ago, the Appalachian Mountains of eastern North America were probably once as high as the Himalaya, but they have been weathered and eroded significantly since the breakup of Pangaea.
Remember that Wegener used the similarity of the mountains on the west and east sides of the Atlantic as evidence for his continental drift hypothesis. The Appalachian mountains formed at a convergent plate boundary as Pangaea came together (figure 15).
Before Pangaea came together, the continents were separated by an ocean where the Atlantic is now. The proto-Atlantic ocean shrank as the Pacific ocean grew. Currently, the Pacific is shrinking as the Atlantic is growing. This supercontinent cycle is responsible for most of the geologic features that we see and many more that are long gone (figure 16).
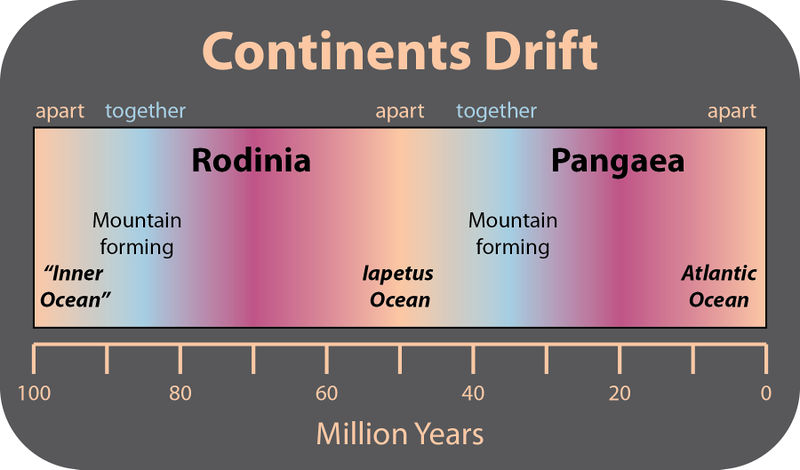
Figure 16. Scientists think that the creation and breakup of a supercontinent takes place about every 500 million years. The supercontinent before Pangaea was Rodinia. A new continent will form as the Pacific ocean disappears.
This animation shows the movement of continents over the past 600 million years beginning with the breakup of Rodinia.
Summary
- Plates of lithosphere move because of convection currents in the mantle. One type of motion is produced by seafloor spreading.
- Plate boundaries can be located by outlining earthquake epicenters.
- Plates interact at three types of plate boundaries: divergent, convergent and transform.
- Most of the Earth’s geologic activity takes place at plate boundaries.
- At a divergent boundary, volcanic activity produces a mid ocean ridge and small earthquakes.
- At a convergent boundary with at least one oceanic plate, an ocean trench, a chain of volcanoes develops and many earthquakes occur.
- At a convergent boundary where both plates are continental, mountain ranges grow and earthquakes are common.
- At a transform boundary, there is a transform fault and massive earthquakes occur but there are no volcanoes.
- Processes acting over long periods of time create Earth’s geographic features.
Developing the Theory
In line with other previous and contemporaneous proposals, in 1912 the meteorologist Alfred Wegener amply described what he called continental drift, expanded in his 1915 book The Origin of Continents and Oceans[1], and the scientific debate started that would end up fifty years later in the theory of plate tectonics. Starting from the idea (also expressed by his forerunners) that the present continents once formed a single land mass (which was called Pangea later on) that drifted apart, thus releasing the continents from the Earth’s mantle and likening them to “icebergs” of low density granite floating on a sea of denser basalt.
Supporting evidence for the idea came from the dove-tailing outlines of South America’s east coast and Africa’s west coast, and from the matching of the rock formations along these edges. Confirmation of their previous contiguous nature also came from the fossil plants Glossopteris and Gangamopteris, and the therapsid or mammal-like reptile Lystrosaurus, all widely distributed over South America, Africa, Antarctica, India and Australia. The evidence for such an erstwhile joining of these continents was patent to field geologists working in the southern hemisphere. The South African Alex du Toit put together a mass of such information in his 1937 publication Our Wandering Continents, and went further than Wegener in recognising the strong links between the Gondwana fragments.
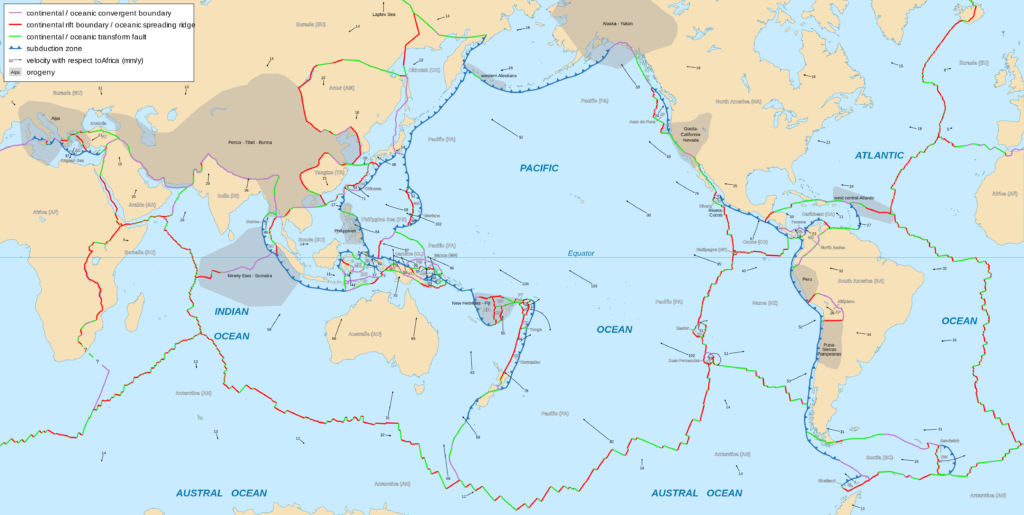
Figure 17. Detailed map showing the tectonic plates with their movement vectors. (Click on the image to open a larger version of the map.)
But without detailed evidence and a force sufficient to drive the movement, the theory was not generally accepted: the Earth might have a solid crust and mantle and a liquid core, but there seemed to be no way that portions of the crust could move around. Distinguished scientists, such as Harold Jeffreys and Charles Schuchert, were outspoken critics of continental drift.
Despite much opposition, the view of continental drift gained support and a lively debate started between “drifters” or “mobilists” (proponents of the theory) and “fixists” (opponents). During the 1920s, 1930s and 1940s, the former reached important milestones proposing that convection currents might have driven the plate movements, and that spreading may have occurred below the sea within the oceanic crust. Concepts close to the elements now incorporated in plate tectonics were proposed by geophysicists and geologists (both fixists and mobilists) like Vening-Meinesz, Holmes, and Umbgrove.
One of the first pieces of geophysical evidence that was used to support the movement of lithospheric plates came from paleomagnetism. This is based on the fact that rocks of different ages show a variable magnetic field direction, evidenced by studies since the mid–nineteenth century. The magnetic north and south poles reverse through time, and, especially important in paleotectonic studies, the relative position of the magnetic north pole varies through time. Initially, during the first half of the twentieth century, the latter phenomenon was explained by introducing what was called “polar wander” (see apparent polar wander), i.e., it was assumed that the north pole location had been shifting through time. An alternative explanation, though, was that the continents had moved (shifted and rotated) relative to the north pole, and each continent, in fact, shows its own “polar wander path”. During the late 1950s it was successfully shown on two occasions that these data could show the validity of continental drift: by Keith Runcorn in a paper in 1956,[2] and by Warren Carey in a symposium held in March 1956.[3]
The second piece of evidence in support of continental drift came during the late 1950s and early 60s from data on the bathymetry of the deep ocean floors and the nature of the oceanic crust such as magnetic properties and, more generally, with the development of marine geology which gave evidence for the association of seafloor spreading along the mid-oceanic ridges and magnetic field reversals, published between 1959 and 1963 by Heezen, Dietz, Hess, Mason, Vine & Matthews, and Morley.
Simultaneous advances in early seismic imaging techniques in and around Wadati-Benioff zones along the trenches bounding many continental margins, together with many other geophysical (e.g. gravimetric) and geological observations, showed how the oceanic crust could disappear into the mantle, providing the mechanism to balance the extension of the ocean basins with shortening along its margins.
All this evidence, both from the ocean floor and from the continental margins, made it clear around 1965 that continental drift was feasible and the theory of plate tectonics, which was defined in a series of papers between 1965 and 1967, was born, with all its extraordinary explanatory and predictive power. The theory revolutionized the Earth sciences, explaining a diverse range of geological phenomena and their implications in other studies such as paleogeography and paleobiology.
Continental Drift
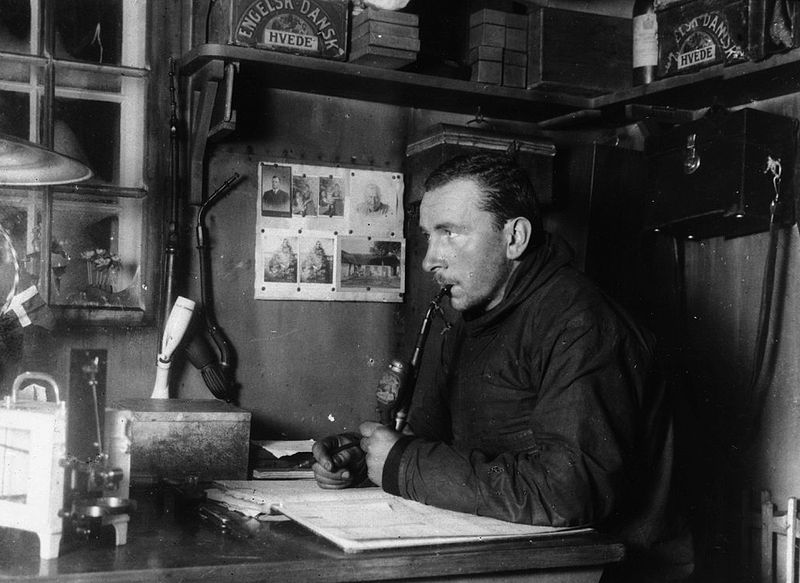
Figure 18. Alfred Wegener in Greenland in the winter of 1912-13.
In the late nineteenth and early twentieth centuries, geologists assumed that the Earth’s major features were fixed, and that most geologic features such as basin development and mountain ranges could be explained by vertical crustal movement, described in what is called the geosynclinal theory. Generally, this was placed in the context of a contracting planet Earth due to heat loss in the course of a relatively short geological time.
It was observed as early as 1596 that the opposite coasts of the Atlantic Ocean—or, more precisely, the edges of the continental shelves—have similar shapes and seem to have once fitted together.
Since that time many theories were proposed to explain this apparent complementarity, but the assumption of a solid Earth made these various proposals difficult to accept.[
The discovery of radioactivity and its associated heating properties in 1895 prompted a re-examination of the apparent age of the Earth. This had previously been estimated by its cooling rate and assumption the Earth’s surface radiated like a black body. Those calculations had implied that, even if it started at red heat, the Earth would have dropped to its present temperature in a few tens of millions of years. Armed with the knowledge of a new heat source, scientists realized that the Earth would be much older, and that its core was still sufficiently hot to be liquid.
By 1915, after having published a first article in 1912, Alfred Wegener was making serious arguments for the idea of continental drift in the first edition of The Origin of Continents and Oceans. In that book (re-issued in four successive editions up to the final one in 1936), he noted how the east coast of South America and the west coast of Africa looked as if they were once attached. Wegener was not the first to note this (Abraham Ortelius, Antonio Snider-Pellegrini, Eduard Suess, Roberto Mantovani and Frank Bursley Taylor preceded him just to mention a few), but he was the first to marshal significant fossil and paleo-topographical and climatological evidence to support this simple observation (and was supported in this by researchers such as Alex du Toit). Furthermore, when the rock strata of the margins of separate continents are very similar it suggests that these rocks were formed in the same way, implying that they were joined initially. For instance, parts of Scotland and Ireland contain rocks very similar to those found in Newfoundland and New Brunswick. Furthermore, the Caledonian Mountains of Europe and parts of the Appalachian Mountainsof North America are very similar in structure and lithology.
However, his ideas were not taken seriously by many geologists, who pointed out that there was no apparent mechanism for continental drift. Specifically, they did not see how continental rock could plow through the much denser rock that makes up oceanic crust. Wegener could not explain the force that drove continental drift, and his vindication did not come until after his death in 1930.
Floating Continents, Paleomagnetism, and Seismicity Zones
As it was observed early that although granite existed on continents, seafloor seemed to be composed of denser basalt, the prevailing concept during the first half of the twentieth century was that there were two types of crust, named “sial” (continental type crust) and “sima” (oceanic type crust). Furthermore, it was supposed that a static shell of strata was present under the continents. It therefore looked apparent that a layer of basalt (sial) underlies the continental rocks.
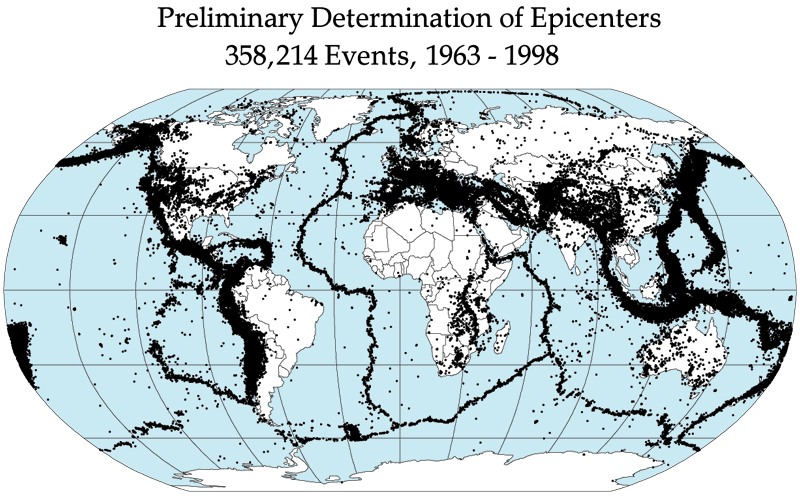
Figure 19. Global earthquake epicenters, 1963–1998
However, based on abnormalities in plumb line deflection by the Andes in Peru, Pierre Bouguer had deduced that less-dense mountains must have a downward projection into the denser layer underneath. The concept that mountains had “roots” was confirmed by George B. Airy a hundred years later, during study of Himalayan gravitation, and seismic studies detected corresponding density variations. Therefore, by the mid-1950s, the question remained unresolved as to whether mountain roots were clenched in surrounding basalt or were floating on it like an iceberg.
During the 20th century, improvements in and greater use of seismic instruments such as seismographs enabled scientists to learn that earthquakes tend to be concentrated in specific areas, most notably along the oceanic trenches and spreading ridges. By the late 1920s, seismologists were beginning to identify several prominent earthquake zones parallel to the trenches that typically were inclined 40–60° from the horizontal and extended several hundred kilometers into the Earth. These zones later became known as Wadati-Benioff zones, or simply Benioff zones, in honor of the seismologists who first recognized them, Kiyoo Wadati of Japan and Hugo Benioff of the United States. The study of global seismicity greatly advanced in the 1960s with the establishment of the Worldwide Standardized Seismograph Network (WWSSN) to monitor the compliance of the 1963 treaty banning above-ground testing of nuclear weapons. The much improved data from the WWSSN instruments allowed seismologists to map precisely the zones of earthquake concentration worldwide.
Meanwhile, debates developed around the phenomena of polar wander. Since the early debates of continental drift, scientists had discussed and used evidence that polar drift had occurred because continents seemed to have moved through different climatic zones during the past. Furthermore, paleomagnetic data had shown that the magnetic pole had also shifted during time. Reasoning in an opposite way, the continents might have shifted and rotated, while the pole remained relatively fixed. The first time the evidence of magnetic polar wander was used to support the movements of continents was in a paper by Keith Runcorn in 1956, and successive papers by him and his students Ted Irving (who was actually the first to be convinced of the fact that paleomagnetism supported continental drift) and Ken Creer.
This was immediately followed by a symposium in Tasmania in March 1956. In this symposium, the evidence was used in the theory of an expansion of the global crust. In this hypothesis the shifting of the continents can be simply explained by a large increase in size of the Earth since its formation. However, this was unsatisfactory because its supporters could offer no convincing mechanism to produce a significant expansion of the Earth. Certainly there is no evidence that the moon has expanded in the past 3 billion years; other work would soon show that the evidence was equally in support of continental drift on a globe with a stable radius.
During the thirties up to the late fifties, works by Vening-Meinesz, Holmes, Umbgrove, and numerous others outlined concepts that were close or nearly identical to modern plate tectonics theory. In particular, the English geologist Arthur Holmes proposed in 1920 that plate junctions might lie beneath the sea, and in 1928 that convection currents within the mantle might be the driving force. Often, these contributions are forgotten because:
- At the time, continental drift was not accepted.
- Some of these ideas were discussed in the context of abandoned fixistic ideas of a deforming globe without continental drift or an expanding Earth.
- They were published during an episode of extreme political and economic instability that hampered scientific communication.
- Many were published by European scientists and at first not mentioned or given little credit in the papers on sea floor spreading published by the American researchers in the 1960s.
Mid-Oceanic Ridge Spreading and Convection
In 1947, a team of scientists led by Maurice Ewing utilizing the Woods Hole Oceanographic Institution’s research vessel Atlantis and an array of instruments, confirmed the existence of a rise in the central Atlantic Ocean, and found that the floor of the seabed beneath the layer of sediments consisted of basalt, not the granite which is the main constituent of continents. They also found that the oceanic crust was much thinner than continental crust. All these new findings raised important and intriguing questions.
The new data that had been collected on the ocean basins also showed particular characteristics regarding the bathymetry. One of the major outcomes of these datasets was that all along the globe, a system of mid-oceanic ridges was detected. An important conclusion was that along this system, new ocean floor was being created, which led to the concept of the “Great Global Rift.” This was described in the crucial paper of Bruce Heezen (1960),[4] which would trigger a real revolution in thinking. A profound consequence of seafloor spreading is that new crust was, and still is, being continually created along the oceanic ridges. Therefore, Heezen advocated the so-called “expanding Earth” hypothesis of S. Warren Carey (see above). So, still the question remained: how can new crust be continuously added along the oceanic ridges without increasing the size of the Earth? In reality, this question had been solved already by numerous scientists during the forties and the fifties, like Arthur Holmes, Vening-Meinesz, Coates and many others: The crust in excess disappeared along what were called the oceanic trenches, where so-called “subduction” occurred. Therefore, when various scientists during the early sixties started to reason on the data at their disposal regarding the ocean floor, the pieces of the theory quickly fell into place.
The question particularly intrigued Harry Hammond Hess, a Princeton University geologist and a Naval Reserve Rear Admiral, and Robert S. Dietz, a scientist with the U.S. Coast and Geodetic Survey who first coined the term seafloor spreading. Dietz and Hess (the former published the same idea one year earlier in Nature,[5] but priority belongs to Hess who had already distributed an unpublished manuscript of his 1962 article by 1960)[6] were among the small handful who really understood the broad implications of sea floor spreading and how it would eventually agree with the, at that time, unconventional and unaccepted ideas of continental drift and the elegant and mobilistic models proposed by previous workers like Holmes.
In the same year, Robert R. Coats of the U.S. Geological Survey described the main features of island arc subduction in the Aleutian Islands. His paper, though little noted (and even ridiculed) at the time, has since been called “seminal” and “prescient.” In reality, it actually shows that the work by the European scientists on island arcs and mountain belts performed and published during the 1930s up until the 1950s was applied and appreciated also in the United States.
If the Earth’s crust was expanding along the oceanic ridges, Hess and Dietz reasoned like Holmes and others before them, it must be shrinking elsewhere. Hess followed Heezen, suggesting that new oceanic crust continuously spreads away from the ridges in a conveyor belt–like motion. And, using the mobilistic concepts developed before, he correctly concluded that many millions of years later, the oceanic crust eventually descends along the continental margins where oceanic trenches—very deep, narrow canyons—are formed, e.g. along the rim of the Pacific Ocean basin. The important step Hess made was that convection currents would be the driving force in this process, arriving at the same conclusions as Holmes had decades before with the only difference that the thinning of the ocean crust was performed using Heezen’s mechanism of spreading along the ridges. Hess therefore concluded that the Atlantic Ocean was expanding while the Pacific Ocean was shrinking. As old oceanic crust is “consumed” in the trenches (like Holmes and others, he thought this was done by thickening of the continental lithosphere, not, as now understood, by underthrusting at a larger scale of the oceanic crust itself into the mantle), new magma rises and erupts along the spreading ridges to form new crust. In effect, the ocean basins are perpetually being “recycled,” with the creation of new crust and the destruction of old oceanic lithosphere occurring simultaneously. Thus, the new mobilistic concepts neatly explained why the Earth does not get bigger with sea floor spreading, why there is so little sediment accumulation on the ocean floor, and why oceanic rocks are much younger than continental rocks.
Magnetic Striping
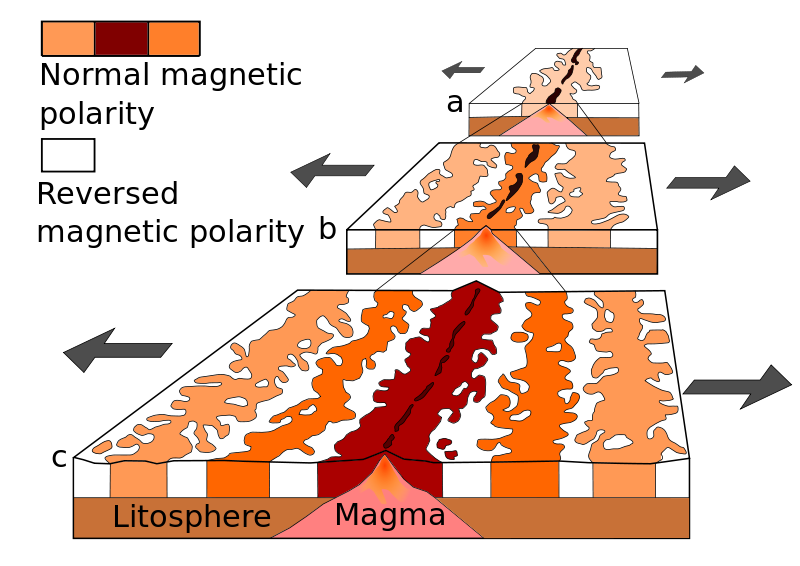
Figure 20. Seafloor magnetic striping
Beginning in the 1950s, scientists like Victor Vacquier, using magnetic instruments (magnetometers) adapted from airborne devices developed during World War II to detect submarines, began recognizing odd magnetic variations across the ocean floor. This finding, though unexpected, was not entirely surprising because it was known that basalt—the iron-rich, volcanic rock making up the ocean floor—contains a strongly magnetic mineral (magnetite) and can locally distort compass readings. This distortion was recognized by Icelandic mariners as early as the late eighteenth century. More important, because the presence of magnetite gives the basalt measurable magnetic properties, these newly discovered magnetic variations provided another means to study the deep ocean floor. When newly formed rock cools, such magnetic materials recorded the Earth’s magnetic field at the time.
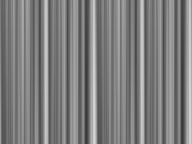
Figure 21. A demonstration of magnetic striping. (The darker the color is, the closer it is to normal polarity)
As more and more of the seafloor was mapped during the 1950s, the magnetic variations turned out not to be random or isolated occurrences, but instead revealed recognizable patterns. When these magnetic patterns were mapped over a wide region, the ocean floor showed a zebra-like pattern: one stripe with normal polarity and the adjoining stripe with reversed polarity. The overall pattern, defined by these alternating bands of normally and reversely polarized rock, became known as magnetic striping, and was published by Ron G. Mason and co-workers in 1961, who did not find, though, an explanation for these data in terms of sea floor spreading, like Vine, Matthews and Morley a few years later.
The discovery of magnetic striping called for an explanation. In the early 1960s scientists such as Heezen, Hess and Dietz had begun to theorise that mid-ocean ridges mark structurally weak zones where the ocean floor was being ripped in two lengthwise along the ridge crest (see the previous paragraph). New magma from deep within the Earth rises easily through these weak zones and eventually erupts along the crest of the ridges to create new oceanic crust. This process, at first denominated the “conveyer belt hypothesis” and later called seafloor spreading, operating over many millions of years continues to form new ocean floor all across the 50,000 km-long system of mid-ocean ridges.
Only four years after the maps with the “zebra pattern” of magnetic stripes were published, the link between sea floor spreading and these patterns was correctly placed, independently by Lawrence Morley, and by Fred Vine and Drummond Matthews, in 1963, now called the Vine-Matthews-Morley hypothesis. This hypothesis linked these patterns to geomagnetic reversals and was supported by several lines of evidence:
- the stripes are symmetrical around the crests of the mid-ocean ridges; at or near the crest of the ridge, the rocks are very young, and they become progressively older away from the ridge crest;
- the youngest rocks at the ridge crest always have present-day (normal) polarity;
- stripes of rock parallel to the ridge crest alternate in magnetic polarity (normal-reversed-normal, etc.), suggesting that they were formed during different epochs documenting the (already known from independent studies) normal and reversal episodes of the Earth’s magnetic field.
By explaining both the zebra-like magnetic striping and the construction of the mid-ocean ridge system, the seafloor spreading hypothesis (SFS) quickly gained converts and represented another major advance in the development of the plate-tectonics theory. Furthermore, the oceanic crust now came to be appreciated as a natural “tape recording” of the history of the geomagnetic field reversals (GMFR) of the Earth’s magnetic field. Today, extensive studies are dedicated to the calibration of the normal-reversal patterns in the oceanic crust on one hand and known timescales derived from the dating of basalt layers in sedimentary sequences (magnetostratigraphy) on the other, to arrive at estimates of past spreading rates and plate reconstructions.
Definition and Refining of the Theory
After all these considerations, Plate Tectonics (or, as it was initially called “New Global Tectonics”) became quickly accepted in the scientific world, and numerous papers followed that defined the concepts:
- In 1965, Tuzo Wilson who had been a promotor of the sea floor spreading hypothesis and continental drift from the very beginning added the concept of transform faults to the model, completing the classes of fault types necessary to make the mobility of the plates on the globe work out.
- A symposium on continental drift was held at the Royal Society of London in 1965 which must be regarded as the official start of the acceptance of plate tectonics by the scientific community, and which abstracts are issued as Blacket, Bullard & Runcorn (1965). In this symposium, Edward Bullard and co-workers showed with a computer calculation how the continents along both sides of the Atlantic would best fit to close the ocean, which became known as the famous “Bullard’s Fit”.
- In 1966 Wilson published the paper that referred to previous plate tectonic reconstructions, introducing the concept of what is now known as the “Wilson Cycle.”
- In 1967, at the American Geophysical Union’s meeting, W. Jason Morgan proposed that the Earth’s surface consists of 12 rigid plates that move relative to each other.
- Two months later, Xavier Le Pichon published a complete model based on 6 major plates with their relative motions, which marked the final acceptance by the scientific community of plate tectonics.
- In the same year, McKenzie and Parker independently presented a model similar to Morgan’s using translations and rotations on a sphere to define the plate motions.
Check Your Understanding
Which characteristics describe divergent boundaries?
- deep sea trenches, the destruction of the seafloor crust, and subduction
- crust being neither created nor destroyed and shearing motion
- plates moving apart, the creation of new seafloor, and the Mid Ocean Ridge
Show Answer
plates moving apart, the creation of new seafloor, and the Mid Ocean Ridge
Candela Citations
- Introduction to The Theory of Plate Tectonics. Authored by: Kimberly Schulte and Lumen Learning. Provided by: Lumen Learning. License: CC BY: Attribution
- 6.4: Theory of Plate Tectonics. Provided by: CK-12. Located at: http://www.ck12.org/book/CK-12-Earth-Science-For-High-School/section/6.4/. License: CC BY-NC: Attribution-NonCommercial
- Plate tectonics. Provided by: Wikipedia. Located at: https://en.wikipedia.org/wiki/Plate_tectonics#Development_of_the_theory. License: CC BY-SA: Attribution-ShareAlike
- Wegener, Alfred (1929). Die Entstehung der Kontinente und Ozeane (4 ed.). Braunschweig: Friedrich Vieweg & Sohn Akt. Ges. ↵
- Runcorn, S.K. (1956). "Paleomagnetic comparisons between Europe and North America". Proceedings, Geological Association of Canada 8 (1088): 7785. ↵
- Carey, S. W. (1958). "The tectonic approach to continental drift." In Carey, S.W. Continental Drift—A symposium, held in March 1956. Hobart: Univ. of Tasmania. pp. 177–363. Expanding Earth from p. 311 to p. 349. ↵
- Heezen, B. (1960). "The rift in the ocean floor." Scientific American 203 (4): 98–110. doi: 10.1038/scientificamerican1060-98. ↵
- Dietz, Robert S. (June 1961). "Continent and Ocean Basin Evolution by Spreading of the Sea Floor". Nature 190 (4779): 854–857. ↵
- Hess, H. H. (November 1962). "History of Ocean Basins" (PDF). In A. E. J. Engel, Harold L. James, and B. F. Leonard. Petrologic studies: a volume to honor of A. F. Buddington. Boulder, CO: Geological Society of America. pp. 599–620. ↵