What you’ll learn to do: Classify different types of atomic bonds
When atoms bond together, they create molecules: a sodium atom bonds with a chlorine atom to create salt (sodium chloride), two hydrogen atoms bond with an oxygen atom to create water (hydrogen dioxide). However, not all atomic bonds are the same; in fact salt and water are created with two very different types of bonds (ionic and polar covalent bonds respectively).
The different types of bonds (ionic, polar covalent, and non-polar covalent bonds) behave differently, and these differences have an impact on the molecules they create. Understanding the types of bonds that create living things can help us understand those living things themselves.
Learning Outcomes
- Define the octet rule and its role in chemical bonds
- Describe the characteristics of ionic bonds and identify common ions
- Describe the characteristics of covalent bonds and differentiate between polar and nonpolar bonds
- Model a Hydrogen bond and identify its unique qualities
- Model van der Waals interactions identify their unique qualities
- Describe the properties of water that are critical to maintaining life
Chemical Bonding
Not all elements have enough electrons to fill their outermost shells, but an atom is at its most stable when all of the electron positions in the outermost shell are filled. Because of these vacancies in the outermost shells, we see the formation of chemical bonds, or interactions between two or more of the same or different elements that result in the formation of molecules. To achieve greater stability, atoms will tend to completely fill their outer shells and will bond with other elements to accomplish this goal by sharing electrons, accepting electrons from another atom, or donating electrons to another atom. Because the outermost shells of the elements with low atomic numbers (up to calcium, with atomic number 20) can hold eight electrons, this is referred to as the octet rule. An element can donate, accept, or share electrons with other elements to fill its outer shell and satisfy the octet rule.
An early model of the atom was developed in 1913 by the Danish scientist Niels Bohr (1885–1962). The Bohr model shows the atom as a central nucleus containing protons and neutrons, with the electrons in circular electron shells at specific distances from the nucleus, similar to planets orbiting around the sun. Each electron shell has a different energy level, with those shells closest to the nucleus being lower in energy than those farther from the nucleus. By convention, each shell is assigned a number and the symbol n—for example, the electron shell closest to the nucleus is called 1n. In order to move between shells, an electron must absorb or release an amount of energy corresponding exactly to the difference in energy between the shells. For instance, if an electron absorbs energy from a photon, it may become excited and move to a higher-energy shell; conversely, when an excited electron drops back down to a lower-energy shell, it will release energy, often in the form of heat.
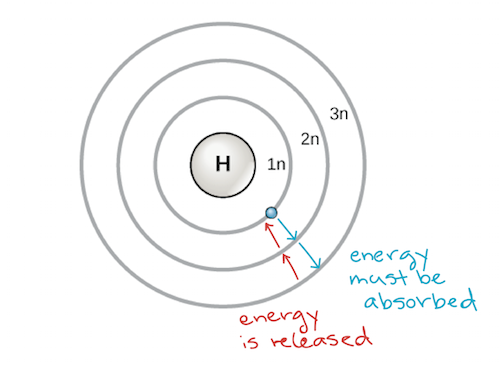
Bohr model of an atom, showing energy levels as concentric circles surrounding the nucleus. Energy must be added to move an electron outward to a higher energy level, and energy is released when an electron falls down from a higher energy level to a closer-in one. Image credit: modified from OpenStax Biology
Atoms, like other things governed by the laws of physics, tend to take on the lowest-energy, most stable configuration they can. Thus, the electron shells of an atom are populated from the inside out, with electrons filling up the low-energy shells closer to the nucleus before they move into the higher-energy shells further out. The shell closest to the nucleus, 1n, can hold two electrons, while the next shell, 2n, can hold eight, and the third shell, 3n, can hold up to eighteen.
The number of electrons in the outermost shell of a particular atom determines its reactivity, or tendency to form chemical bonds with other atoms. This outermost shell is known as the valence shell, and the electrons found in it are called valence electrons. In general, atoms are most stable, least reactive, when their outermost electron shell is full. Most of the elements important in biology need eight electrons in their outermost shell in order to be stable, and this rule of thumb is known as the octet rule. Some atoms can be stable with an octet even though their valence shell is the 3n shell, which can hold up to 18 electrons. We will explore the reason for this when we discuss electron orbitals below.
Examples of some neutral atoms and their electron configurations are shown below. In this table, you can see that helium has a full valence shell, with two electrons in its first and only, 1n, shell. Similarly, neon has a complete outer 2n shell containing eight electrons. These electron configurations make helium and neon very stable. Although argon does not technically have a full outer shell, since the 3n shell can hold up to eighteen electrons, it is stable like neon and helium because it has eight electrons in the 3n shell and thus satisfies the octet rule. In contrast, chlorine has only seven electrons in its outermost shell, while sodium has just one. These patterns do not fill the outermost shell or satisfy the octet rule, making chlorine and sodium reactive, eager to gain or lose electrons to reach a more stable configuration.
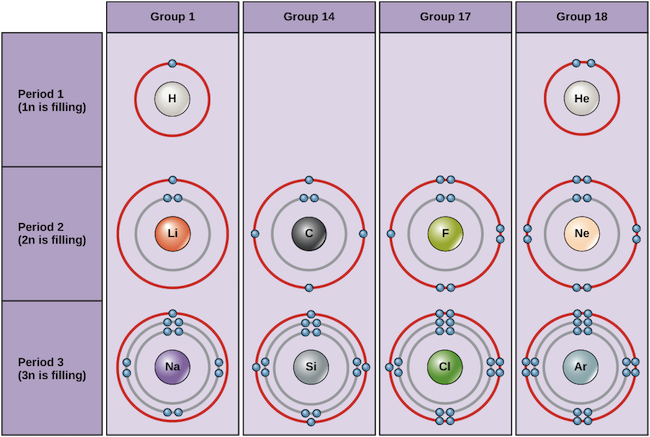
Bohr diagrams of various elements Image credit: OpenStax Biology
Electron configurations and the periodic table
Elements are placed in order on the periodic table based on their atomic number, how many protons they have. In a neutral atom, the number of electrons will equal the number of protons, so we can easily determine electron number from atomic number. In addition, the position of an element in the periodic table—its column, or group, and row, or period—provides useful information about how those electrons are arranged.
If we consider just the first three rows of the table, which include the major elements important to life, each row corresponds to the filling of a different electron shell: helium and hydrogen place their electrons in the 1n shell, while second-row elements like Li start filling the 2n shell, and third-row elements like Na continue with the 3n shell. Similarly, an element’s column number gives information about its number of valence electrons and reactivity. In general, the number of valence electrons is the same within a column and increases from left to right within a row. Group 1 elements have just one valence electron and group 18 elements have eight, except for helium, which has only two electrons total. Thus, group number is a good predictor of how reactive each element will be:
- Helium (He), neon (Ne), and argon (Ar), as group 18 elements, have outer electron shells that are full or satisfy the octet rule. This makes them highly stable as single atoms. Because of their non-reactivity, they are called the inert gases or noble gases.
- Hydrogen (H), lithium (Li), and sodium (Na), as group 1 elements, have just one electron in their outermost shells. They are unstable as single atoms, but can become stable by losing or sharing their one valence electron. If these elements fully lose an electron—as Li and Na typically do—they become positively charged ions: Li+, Na+.
- Fluorine (F) and chlorine (Cl), as group 17 elements, have seven electrons in their outermost shells. They tend to achieve a stable octet by taking an electron from other atoms, becoming negatively charged ions: F− and Cl−.
- Carbon (C), as a group 14 element, has four electrons in its outer shell. Carbon typically shares electrons to achieve a complete valence shell, forming bonds with multiple other atoms.
Thus, the columns of the periodic table reflect the number of electrons found in each element’s valence shell, which in turn determines how the element will react.
Ionic Bonds
Some atoms are more stable when they gain or lose an electron (or possibly two) and form ions. This fills their outermost electron shell and makes them energetically more stable. Because the number of electrons does not equal the number of protons, each ion has a net charge. Cations are positive ions that are formed by losing electrons. Negative ions are formed by gaining electrons and are called anions. Anions are designated by their elemental name being altered to end in “-ide”: the anion of chlorine is called chloride, and the anion of sulfur is called sulfide, for example.
This movement of electrons from one element to another is referred to as electron transfer. As Figure 1 illustrates, sodium (Na) only has one electron in its outer electron shell. It takes less energy for sodium to donate that one electron than it does to accept seven more electrons to fill the outer shell. If sodium loses an electron, it now has 11 protons, 11 neutrons, and only 10 electrons, leaving it with an overall charge of +1. It is now referred to as a sodium ion. Chlorine (Cl) in its lowest energy state (called the ground state) has seven electrons in its outer shell. Again, it is more energy-efficient for chlorine to gain one electron than to lose seven. Therefore, it tends to gain an electron to create an ion with 17 protons, 17 neutrons, and 18 electrons, giving it a net negative (–1) charge. It is now referred to as a chloride ion. In this example, sodium will donate its one electron to empty its shell, and chlorine will accept that electron to fill its shell. Both ions now satisfy the octet rule and have complete outermost shells. Because the number of electrons is no longer equal to the number of protons, each is now an ion and has a +1 (sodium cation) or –1 (chloride anion) charge. Note that these transactions can normally only take place simultaneously: in order for a sodium atom to lose an electron, it must be in the presence of a suitable recipient like a chlorine atom.
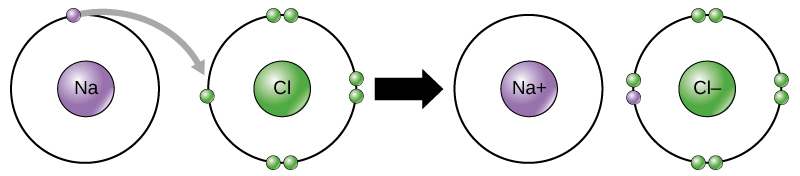
Figure 1. In the formation of an ionic compound, metals lose electrons and nonmetals gain electrons to achieve an octet. Ionic bonds are formed between ions with opposite charges. For instance, positively charged sodium ions and negatively charged chloride ions bond together to make crystals of sodium chloride, or table salt, creating a crystalline molecule with zero net charge.
Certain salts are referred to in physiology as electrolytes (including sodium, potassium, and calcium), ions necessary for nerve impulse conduction, muscle contractions and water balance. Many sports drinks and dietary supplements provide these ions to replace those lost from the body via sweating during exercise.
Video Review
This video shows how ionic compounds form from anions and cations.
Covalent Bonds
Another way the octet rule can be satisfied is by the sharing of electrons between atoms to form covalent bonds. These bonds are stronger and much more common than ionic bonds in the molecules of living organisms. Covalent bonds are commonly found in carbon-based organic molecules, such as our DNA and proteins. Covalent bonds are also found in inorganic molecules like H2O, CO2, and O2. One, two, or three pairs of electrons may be shared, making single, double, and triple bonds, respectively. The more covalent bonds between two atoms, the stronger their connection. Thus, triple bonds are the strongest.
The strength of different levels of covalent bonding is one of the main reasons living organisms have a difficult time in acquiring nitrogen for use in constructing their molecules, even though molecular nitrogen, N2, is the most abundant gas in the atmosphere. Molecular nitrogen consists of two nitrogen atoms triple bonded to each other and, as with all molecules, the sharing of these three pairs of electrons between the two nitrogen atoms allows for the filling of their outer electron shells, making the molecule more stable than the individual nitrogen atoms. This strong triple bond makes it difficult for living systems to break apart this nitrogen in order to use it as constituents of proteins and DNA.
The formation of water molecules provides an example of covalent bonding. The hydrogen and oxygen atoms that combine to form water molecules are bound together by covalent bonds. The electron from the hydrogen splits its time between the incomplete outer shell of the hydrogen atoms and the incomplete outer shell of the oxygen atoms. To completely fill the outer shell of oxygen, which has six electrons in its outer shell but which would be more stable with eight, two electrons (one from each hydrogen atom) are needed: hence the well-known formula H2O. The electrons are shared between the two elements to fill the outer shell of each, making both elements more stable.
Watch this video for another explanation of covalent bonds and how they form:
Polar and Nonpolar Covalent Bonds
There are two types of covalent bonds: polar and nonpolar. Nonpolar covalent bonds form between two atoms of the same element or between different elements that share the electrons equally. For example, an oxygen atom can bond with another oxygen atom to fill their outer shells. This association is nonpolar because the electrons will be equally distributed between each oxygen atom. Two covalent bonds form between the two oxygen atoms because oxygen requires two shared electrons to fill its outermost shell. Nitrogen atoms will form three covalent bonds (also called triple covalent) between two atoms of nitrogen because each nitrogen atom needs three electrons to fill its outermost shell. Another example of a nonpolar covalent bond is found in the methane (CH4) molecule. The carbon atom has four electrons in its outermost shell and needs four more to fill it. It gets these four from four hydrogen atoms, each atom providing one. These elements all share the electrons equally, creating four nonpolar covalent bonds.
In a polar covalent bond, the electrons shared by the atoms spend more time closer to one nucleus than to the other nucleus. Because of the unequal distribution of electrons between the different nuclei, a slightly positive (δ+) or slightly negative (δ–) charge develops. The covalent bonds between hydrogen and oxygen atoms in water are polar covalent bonds. The shared electrons spend more time near the oxygen nucleus, giving it a small negative charge, than they spend near the hydrogen nuclei, giving these molecules a small positive charge. Polar covalent bonds form more often when atoms that differ greatly in size share electrons.
Examples of Covalent Bonding
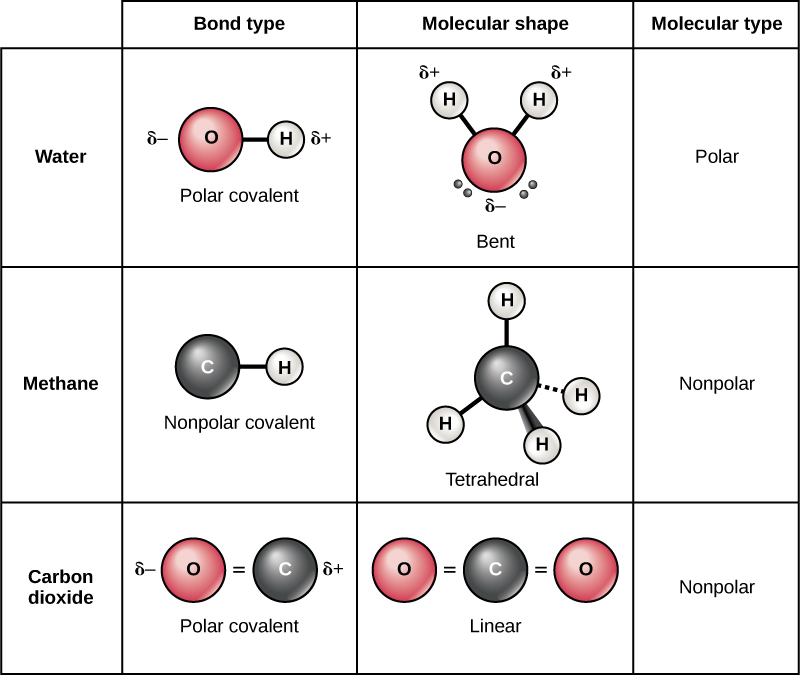
Figure 2. Whether a molecule is polar or nonpolar depends both on bond type and molecular shape. Both water and carbon dioxide have polar covalent bonds, but carbon dioxide is linear, so the partial charges on the molecule cancel each other out.
Video Review
Watch this video for another explanation of covalent bonds and how they form:
Hydrogen Bonds
Ionic and covalent bonds between elements require energy to break. Iconic bonds are not as strong as covalent, which determines their behavior in biological systems. However, not all bonds are ionic or covalent bonds. Weaker bonds can also form between molecules. Two weak bonds that occur frequently are hydrogen bonds and van der Waals interactions. Without these two types of bonds, life as we know it would not exist. Hydrogen bonds provide many of the critical, life-sustaining properties of water and also stabilize the structures of proteins and DNA, the building block of cells.
When polar covalent bonds containing hydrogen form, the hydrogen in that bond has a slightly positive charge because hydrogen’s electron is pulled more strongly toward the other element and away from the hydrogen. Because the hydrogen is slightly positive, it will be attracted to neighboring negative charges. When this happens, a weak interaction occurs between the δ+ of the hydrogen from one molecule and the δ– charge on the more electronegative atoms of another molecule, usually oxygen or nitrogen, or within the same molecule.
Hydrogen Bonding between water molecules
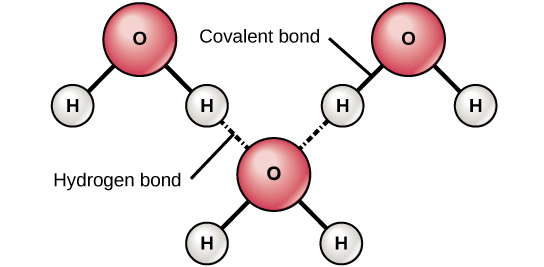
Figure 3. Hydrogen bonds form between slightly positive (δ+) and slightly negative (δ–) charges of polar covalent molecules, such as water.
This interaction is called a hydrogen bond. This type of bond is common and occurs regularly between water molecules. Individual hydrogen bonds are weak and easily broken; however, they occur in very large numbers in water and in organic polymers, creating a major force in combination. Hydrogen bonds are also responsible for zipping together the DNA double helix.
Video Review
van der Waals Interactions
Like hydrogen bonds, van der Waals interactions are weak attractions or interactions between molecules. They are also called inter-molecular forces. They occur between polar, covalently bound atoms in different molecules. Some of these weak attractions are caused by temporary partial charges formed when electrons move around a nucleus. These weak interactions between molecules are important in biological systems and occur based on physical proximity.
Radiology Technician
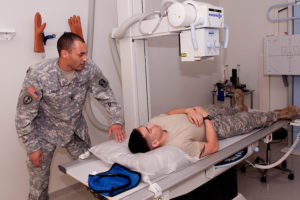
Figure 4. Spc. Arbor L. LaClave practices his spinal X-ray positions utilizing Spc. Justin J. Reichelt, a radiology technician, as his mock patient to practice his skills in the health clinic at Grafenwoehr Training Area.
Have you or anyone you know ever had a magnetic resonance imaging (MRI) scan, a mammogram, or an X-ray? These tests produce images of your soft tissues and organs (as with an MRI or mammogram) or your bones (as happens in an X-ray) by using either radiowaves or special isotopes (radiolabeled or fluorescently labeled) that are ingested or injected into the body. These tests provide data for disease diagnoses by creating images of your organs or skeletal system.
MRI imaging works by subjecting hydrogen nuclei, which are abundant in the water in soft tissues, to fluctuating magnetic fields, which cause them to emit their own magnetic field. This signal is then read by sensors in the machine and interpreted by a computer to form a detailed image.
Some radiography technologists and technicians specialize in computed tomography, MRI, and mammography. They produce films or images of the body that help medical professionals examine and diagnose. Radiologists work directly with patients, explaining machinery, preparing them for exams, and ensuring that their body or body parts are positioned correctly to produce the needed images. Physicians or radiologists then analyze the test results.
Radiography technicians can work in hospitals, doctors’ offices, or specialized imaging centers. Training to become a radiography technician happens at hospitals, colleges, and universities that offer certificates, associate’s degrees, or bachelor’s degrees in radiography.
Why Life Depends on Water
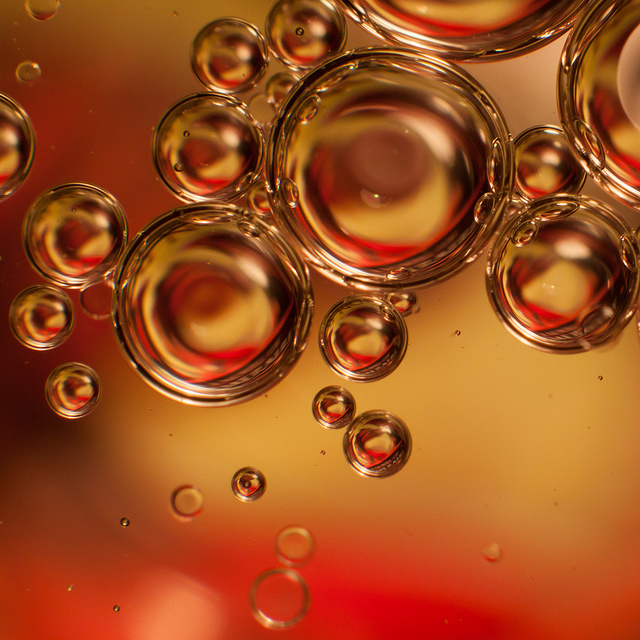
Figure 5. As this image of oil and water shows, oil is a nonpolar compound and, hence, will not dissolve in water. Oil and water do not mix.
Do you ever wonder why scientists spend time looking for water on other planets? It is because water is essential to life; even minute traces of it on another planet can indicate that life could or did exist on that planet. Water is one of the more abundant molecules in living cells and the one most critical to life as we know it. Approximately 60–70 percent of your body is made up of water. Without it, life simply would not exist.
Water Is Polar
The hydrogen and oxygen atoms within water molecules form polar covalent bonds. The shared electrons spend more time associated with the oxygen atom than they do with hydrogen atoms. There is no overall charge to a water molecule, but there is a slight positive charge on each hydrogen atom and a slight negative charge on the oxygen atom. Because of these charges, the slightly positive hydrogen atoms repel each other and form the unique shape seen in Figure 6. Each water molecule attracts other water molecules because of the positive and negative charges in the different parts of the molecule. Water also attracts other polar molecules (such as sugars), forming hydrogen bonds. When a substance readily forms hydrogen bonds with water, it can dissolve in water and is referred to as hydrophilic (“water-loving”). Hydrogen bonds are not readily formed with nonpolar substances like oils and fats (Figure 5). These nonpolar compounds are hydrophobic (“water-fearing”) and will not dissolve in water.
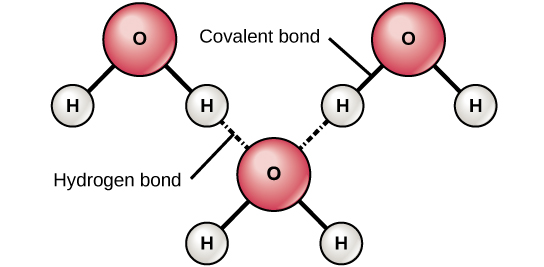
Figure 6. Hydrogen bonds form between slightly positive (δ+) and slightly negative (δ–) charges of polar covalent molecules, such as water.
Water Stabilizes Temperature
The hydrogen bonds in water allow it to absorb and release heat energy more slowly than many other substances. Temperature is a measure of the motion (kinetic energy) of molecules. As the motion increases, energy is higher and thus temperature is higher. Water absorbs a great deal of energy before its temperature rises. Increased energy disrupts the hydrogen bonds between water molecules. Because these bonds can be created and disrupted rapidly, water absorbs an increase in energy and temperature changes only minimally. This means that water moderates temperature changes within organisms and in their environments. As energy input continues, the balance between hydrogen-bond formation and destruction swings toward the destruction side. More bonds are broken than are formed. This process results in the release of individual water molecules at the surface of the liquid (such as a body of water, the leaves of a plant, or the skin of an organism) in a process called evaporation. Evaporation of sweat, which is 90 percent water, allows for cooling of an organism, because breaking hydrogen bonds requires an input of energy and takes heat away from the body.
Conversely, as molecular motion decreases and temperatures drop, less energy is present to break the hydrogen bonds between water molecules. These bonds remain intact and begin to form a rigid, lattice-like structure (e.g., ice) (Figure 7a). When frozen, ice is less dense than liquid water (the molecules are farther apart). This means that ice floats on the surface of a body of water (Figure 7b). In lakes, ponds, and oceans, ice will form on the surface of the water, creating an insulating barrier to protect the animal and plant life beneath from freezing in the water. If this did not happen, plants and animals living in water would freeze in a block of ice and could not move freely, making life in cold temperatures difficult or impossible.
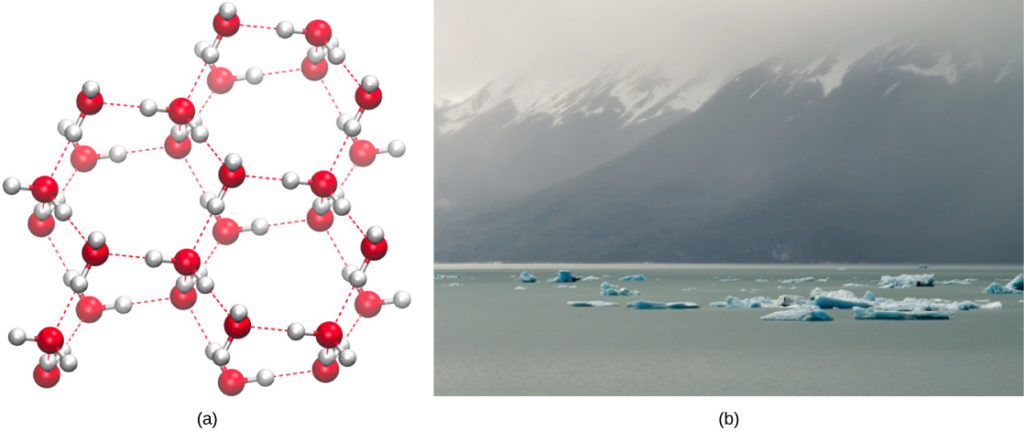
Figure 7. (a) The lattice structure of ice makes it less dense than the freely flowing molecules of liquid water. Ice’s lower density enables it to (b) float on water. (credit a: modification of work by Jane Whitney; credit b: modification of work by Carlos Ponte)
Water Is an Excellent Solvent
Because water is polar, with slight positive and negative charges, ionic compounds and polar molecules can readily dissolve in it. Water is, therefore, what is referred to as a solvent—a substance capable of dissolving another substance. The charged particles will form hydrogen bonds with a surrounding layer of water molecules. This is referred to as a sphere of hydration and serves to keep the particles separated or dispersed in the water. In the case of table salt (NaCl) mixed in water (Figure 8), the sodium and chloride ions separate, or dissociate, in the water, and spheres of hydration are formed around the ions.
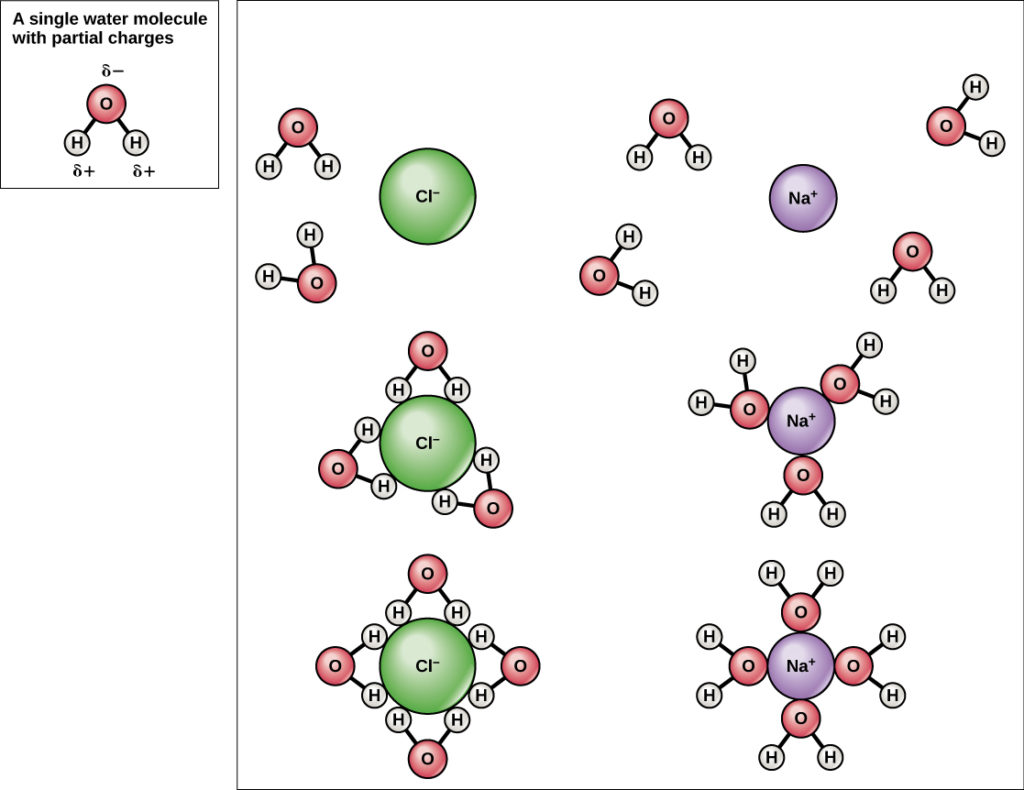
Figure 8. When table salt (NaCl) is mixed in water, spheres of hydration form around the ions.
A positively charged sodium ion is surrounded by the partially negative charges of oxygen atoms in water molecules. A negatively charged chloride ion is surrounded by the partially positive charges of hydrogen atoms in water molecules. These spheres of hydration are also referred to as hydration shells. The polarity of the water molecule makes it an effective solvent and is important in its many roles in living systems.
Water Is Cohesive
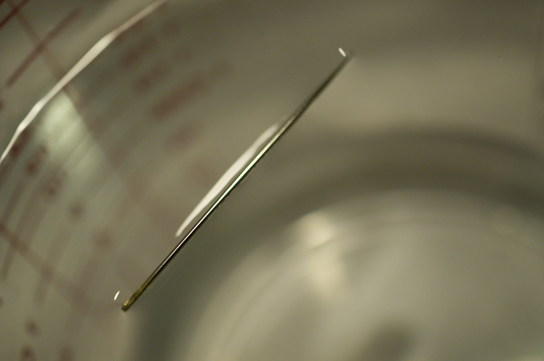
Figure 9. The weight of a needle on top of water pulls the surface tension downward; at the same time, the surface tension of the water is pulling it up, suspending the needle on the surface of the water and keeping it from sinking. Notice the indentation in the water around the needle. (credit: Cory Zanker)
Have you ever filled up a glass of water to the very top and then slowly added a few more drops? Before it overflows, the water actually forms a dome-like shape above the rim of the glass. This water can stay above the glass because of the property of cohesion. In cohesion, water molecules are attracted to each other (because of hydrogen bonding), keeping the molecules together at the liquid-air (gas) interface, although there is no more room in the glass. Cohesion gives rise to surface tension, the capacity of a substance to withstand rupture when placed under tension or stress. When you drop a small scrap of paper onto a droplet of water, the paper floats on top of the water droplet, although the object is denser (heavier) than the water. This occurs because of the surface tension that is created by the water molecules. Cohesion and surface tension keep the water molecules intact and the item floating on the top. It is even possible to “float” a steel needle on top of a glass of water if you place it gently, without breaking the surface tension (Figure 9).
These cohesive forces are also related to the water’s property of adhesion, or the attraction between water molecules and other molecules. This is observed when water “climbs” up a straw placed in a glass of water. You will notice that the water appears to be higher on the sides of the straw than in the middle. This is because the water molecules are attracted to the straw and therefore adhere to it.
Cohesive and adhesive forces are important for sustaining life. For example, because of these forces, water can flow up from the roots to the tops of plants to feed the plant.
Video Review
Practice Question
Which of the following statements is not true?
- Water is polar.
- Water stabilizes temperature.
- Water is essential for life.
- Water is the most abundant atom in Earth’s atmosphere.
Check Your Understanding
Which type of bond represents a weak chemical bond?
- hydrogen bond
- covalent bond
- ionic bond
Show Answer
hydrogen bond
Candela Citations
- Introduction to Atomic Bonds. Authored by: Shelli Carter and Lumen Learning. Provided by: Lumen Learning. License: CC BY: Attribution
- Concepts of Biology. Provided by: OpenStax CNX. Located at: http://cnx.org/contents/b3c1e1d2-839c-42b0-a314-e119a8aafbdd@9.25. License: CC BY: Attribution. License Terms: Download for free at http://cnx.org/contents/b3c1e1d2-839c-42b0-a314-e119a8aafbdd@9.25
- The periodic table, electron shells, and orbitals. Provided by: Khan Academy. Located at: https://www.khanacademy.org/science/biology/chemistry--of-life/electron-shells-and-orbitals/a/the-periodic-table-electron-shells-and-orbitals-article. License: CC BY-NC-SA: Attribution-NonCommercial-ShareAlike
- Biology. Provided by: OpenStax CNX. Located at: http://cnx.org/contents/185cbf87-c72e-48f5-b51e-f14f21b5eabd@10.8. License: CC BY: Attribution. License Terms: Download for free at http://cnx.org/contents/185cbf87-c72e-48f5-b51e-f14f21b5eabd@10.8
- Radiology Technician. Authored by: Army Medicine. Located at: https://flic.kr/p/a1Jtxm. License: CC BY-SA: Attribution-ShareAlike
- Oil Abstract [247/366]. Authored by: Tim Sackton. Located at: https://flic.kr/p/d6Lq33. License: CC BY-SA: Attribution-ShareAlike
- Ionic Bonding. Authored by: Bozeman Science. Located at: https://youtu.be/hiyTfhjeF_U. License: All Rights Reserved. License Terms: Standard YouTube License
- Ionic and covalent bonding animation. Authored by: Edu2000.org. Located at: https://youtu.be/QqjcCvzWwww. License: All Rights Reserved. License Terms: Standard YouTube License
- Covalent Bonding. Authored by: Bozeman Science. Located at: https://youtu.be/Mo4Vfqt5v2A. License: All Rights Reserved. License Terms: Standard YouTube License
- Water: A Polar Molecule. Authored by: Bozeman Science. Located at: https://youtu.be/iOOvX0jmhJ4. License: All Rights Reserved. License Terms: Standard YouTube License
- Water - Liquid Awesome: Crash Course Biology #2. Authored by: CrashCourse. Located at: https://youtu.be/HVT3Y3_gHGg. License: All Rights Reserved. License Terms: Standard YouTube License