Key Concepts
After completing this chapter, you will be able to:
- Explain the factors that allow cities to exist and support their human population.
- Describe recent trends of rapid urbanization.
- Describe the structural and functional characteristics of the urban ecosystem.
- Define the concept of an ecological footprint.
- Explain the essential elements of urban planning and how it has affected land-use in cities.
- List the major kinds of urban pollution and describe their causes and recent trends in cities.
- Describe the methods of waste management.
- Describe the management of solid waste and sewage in urban areas.
- Explain the major elements of urban biodiversity and how it can be better managed through the naturalization of habitats.
Introduction
The Development of Urban Areas
Urban areas are cities, towns, and other places where people live in a compact population. Most urban people are engaged in economic activities that function efficiently in dense populations, such as commerce, education, manufacturing, and services. In contrast, resource-based economic activities, such as cultivating food and harvesting minerals, fossil fuels, timber, or wildlife, occur primarily in rural areas.
Urban areas import energy, food, and materials from the local countryside, as well as from more distant regions of their country, in addition to other nations around the world in an increasingly global commerce. The resource-related connections between cities and rural areas exist at all spatial scales (local, regional, and global) and are an integral but often insufficiently appreciated aspect of urban ecology.
The development of dense habitations (initially small villages) during human cultural evolution began about 10-thousand years ago, when early agricultural practices allowed the production of local surpluses of food (harvests that exceeded the subsistence needs of the farmers themselves). The excess food encouraged the development of specialized occupations that were most efficiently performed in a central place such as a village or town. New societal activities included organized religion and political systems, artisanal manufacturing, and the means of administering these over wide areas through hierarchical economic, political, religious, and military structures.
The first villages were probably supported by both settled agriculture and local hunting-and-gathering activities. By about 9,000 years ago, small farming villages were relatively widespread in some regions, particularly in the Fertile Crescent of the Middle East (see Chapter 13). By 8,500 years ago, that region boasted sizable walled towns. Similar developments also occurred in China and probably elsewhere in southern Asia.
From these humble beginnings, urbanization of the human population has proceeded apace, and especially rapidly during the past century. Only about 2.5% of the human population lived in towns and cities in 1800, and 5-10% in 1900. Today, about half of the human population lives in urban places, and this is predicted to rise to two-thirds by 2025 (or about 5 billion people). About 90% of this increase in urban populations will occur in less-developed countries (see Chapter 10). Globally, the urban population is now growing about four times as quickly as that of rural areas, largely because of the migration of huge numbers of people from the countryside to built-up areas. In the U.S. in 1860, about 20% of the population lived in towns and cities in, increasing to 40% in 1900, 64% in 1950, and 81% in 2010 (U.S. Census, 2010).
In 1750, few cities supported more than 50-thousand people – London was the only one in England, and there were none in North America. In 1830, New York and Philadelphia were the only U.S. cities with a population greater than 100-thousand. By 1910, however, 52 cities in North America supported more than 100-thousand people (New York was the largest, with more than 1 million). In 1950, New York and Tokyo were the only two megacities in the world (that is, having a population greater than 10 million). In 2014, there were 537 cities supporting more than 1 million people, including 35 megacities, of which 29 were in the developing world (Brinkhoff, 2015). The largest megacities are listed in Table 10.1.
No effective discussion of urban population policies has occurred in any country. The key issues are how large should cities be in order to provide people with safe and clean places to live, while also supplying the goods and services that urban areas can deliver most effectively? If the largest cities are considered overpopulated, how can continued population growth be discouraged? Can people be encouraged to move to smaller centers? These and other questions related to urban population policies are extremely controversial, but all will have to be addressed.
Urban Ecosystems
Any urbanized area can be viewed as being an ecosystem, because it has the following ecological attributes:
- A need for enormous inputs of energy and materials to sustain its human population and its diverse economic activities, and to maintain its structure and grow
- A complex metabolism, including well-developed webs of transfer, processing, and storage of materials, energy, and information among interacting organisms and economic sectors
- Immense outputs of heat and other waste materials, which are disposed of in surrounding ecosystems, causing pollution and other environmental problems
Of course, the habitats of cities and towns are very strongly influenced by human activities. Collectively they comprise an urban–industrial techno-ecosystem (Chapter 8). The structure of this anthropogenic ecosystem is dominated by the businesses, dwellings, factories, roads, and other infrastructure of the human economy, while also supporting manicured green space as well as remnants of natural habitats in parks and other less-developed spaces. Although humans are the dominant species in the urban ecosystem, many other species are also supported, most of which are not native to the region (Image 28.1). Important ecological functions also occur within the urban ecosystem, such as biological productivity and water and nutrient cycling, but these processes are greatly influenced by humans.
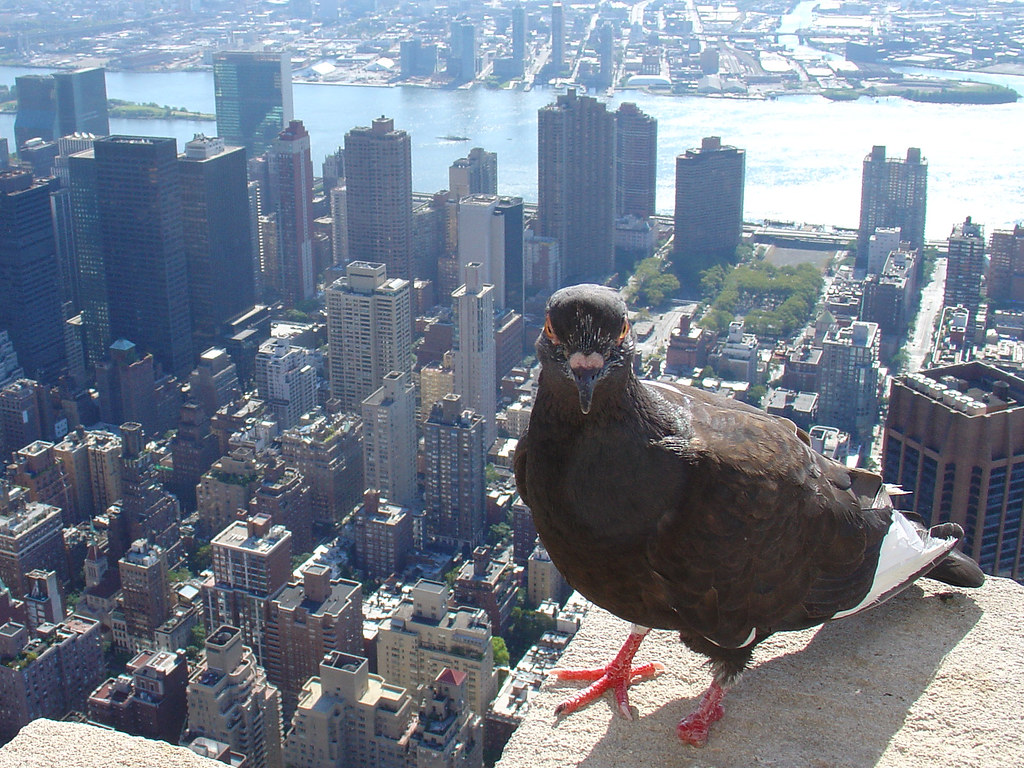
Image 28.1. Urban environments, like all other ecosystems, are composed of organisms influenced by abiotic factors and characterized by nutrient cycles and energy flows. What distinguishes urban environments is the large number of humans and the drastic influence they exert on physical spatial composition, as well as the disruption of otherwise typical ecological cycles, such as the removal and displacement of both organic and inorganic waste. Additionally, urban ecosystems are typically centers of impermeable surfaces, machinery, the consumption of resources, and the resulting heat island effect. However, some organisms have found it beneficial to live in such environments where they are suited to live alongside humans and the influence we exert. Some examples of such animals include rats, raccoons, squirrels, pigeons, stray cats, etc. Source: “One-Legged Pigeon” by ZeroOne is licensed under CC BY-SA 2.0.
All urban areas are intrinsically dependent on surrounding ecosystems to provide them with necessary resources and to assimilate wastes that are generated. The ecological footprint (or eco-footprint) of an urban population is the area of ecoscape (landscape and seascape) that is needed to supply the necessary food, energy, materials, waste disposal, and other crucial goods and services. As a global average, the average human has an eco-footprint of about 2.7 hectares, but there are only 1.8 ha of bioproductive land and water on Earth (Ewing et al., 2010). This means that the human enterprise has already overshot global biocapacity by 30%, and is now operating on an unsustainable basis by depleting the remaining stocks of “natural capital”. Note that, in the context of ecological footprints, these data are measures in “global hectares” (gha), which represent the average productivity of all bio-productive habitats on Earth or in a country, including agricultural land, forests, and fishing grounds, but not including desert, glaciers, or the open ocean.
An average American has an ecological footprint of about 8.04 global hectares (2017 data; Figure 28.1). This is the eighth-most intensive national per-capita footprint in the world, after Qatar (14.72 gha), Luxembourg (12.79), the United Arab Emirates (8.95 gha), Bahrain (8.66), Trinidad and Tobago (8.23 gha), Canada (8.08 gha), and Mongolia (8.05 gha).
Here are some additional comparisons among wealthier countries: Australia (7.27 gha), Kuwait (8.03 gha), Ireland (5.01 gha), Norway (5.78 gha), France (4.6 gha), Germany (4.7gha), United Kingdom (4.2 gha), and Japan (4.65 gha). The rapidly-growing economies include Russia (5.48 gha), Brazil (2.81 gha), China (3.71 gha), and India (1.9 gha). Of course, people living in poorer countries have much smaller ecological footprints: Afghanistan (0.66 gha), Bangladesh (0.83 gha), Haiti (0.64 gha), Burundi (0.63 gha), Ethiopia (1.01 gha), Vietnam (2.24 gha), and Peru, (2.15 gha) (Global Footprint Network, 2017).
The major influences on the differences in these ecological footprints are related to the intensity of energy and material use and waste production within the national economies. The major elements of the footprint are related to emissions of carbon dioxide (a greenhouse gas; this is known as the “carbon footprint”) and activities associated with harvesting or otherwise damaging forests and engaging in agricultural and fishing activities.
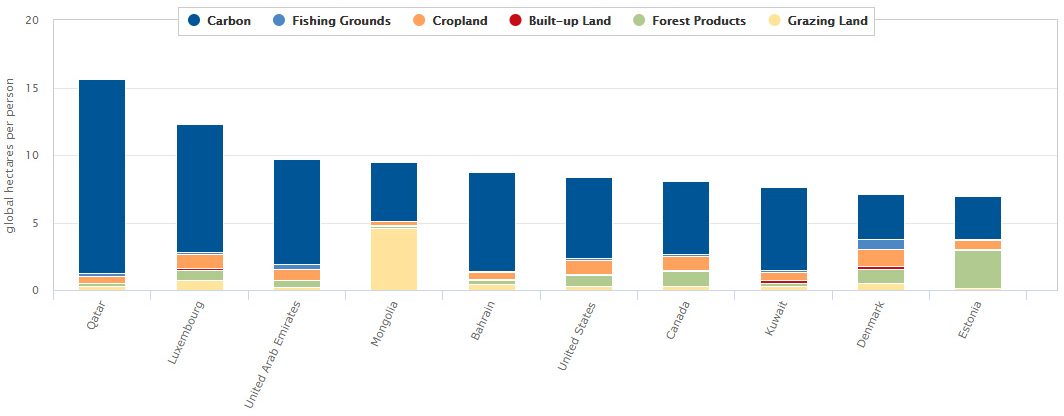
Figure 28.1. Top Ten Countries with Largest Ecological Footprint per Person. The data is based on the relative contribution of each category to the per-capita ecological footprint. Source: Data modified from Global Footprint Network (2017).
Based on the American national ecological footprint of 8.04 gha/person, the ecological footprints of the U.S.’s five largest cities are the following (data are in millions of hectares):
- New York: 67.2
- Los Angeles: 32.0
- Chicago: 21.7
- Houston: 18.7
- Phoenix: 13.5
These footprints are about several hundred times larger than the actual areas of these cities. Without such enormous regions to draw upon for resources, these and all other urban areas would be unable to survive.
The Organization of Cities
Urban Planning
Urban ecosystems have extremely complex structures and functions (although not more so than natural ecosystems). To some degree, their development has occurred in an orderly fashion, with certain areas being designated for particular kinds of structures and activities. Urban planning is the active process of designing and organizing the structure and function of cities. As such, urban planning contributes to the information needed by legislators and other decision makers as they develop sensible and efficient siting of the following:
- Buildings, including homes, commercial properties, institutions (such as hospitals and schools), and industrial facilities
- Infrastructure for transportation, utilities, and waste management, such as roads, railways, public-transit routes, electrical transmission and pipeline corridors, sewers and sewage-treatment facilities, and solid-waste disposal areas
- Greenspaces, including playing fields and horticultural and natural-area parks
Well-planned urban areas have relatively pleasant neighborhoods where people live and work. In contrast, poorly planned cities are chaotic, dirty, and unpleasant.
The dominant planning paradigm of the past 60 years has involved the segregation of major land-uses and economic activities into different areas. This kind of strategy has greatly influenced the design of modern cities, including those in U.S. It has resulted in many urban people living in discrete inner-city neighborhoods or more distant suburbs, while shopping in large malls, working in factory or office complexes and industrial parks, and commuting long distances among these land-use types. However, this type of planning has contributed to some important urban problems, including the following:
- The rapid growth of huge, multi-city, urbanized regions (sometimes known as conurbations)
- The inefficient segregation of residences from places of work and commerce
- Long commuting times for workers
- Congested transportation systems
- A decay of neighborhood life
- Environmental problems such as air and water pollution, wasteful use of energy and materials, paving of valuable farmland (many urban areas are located on excellent agricultural land), and losses of natural habitat
Some of these issues are examined in more detail later in this chapter.
The paradigm of widely segregated land-use is now being challenged by the concept of a more integrated “neighborhood” design. This involves the development of relatively compact, self-sufficient communities that contain a mixture of residential and commercial land-uses. In some respects, this harkens back to more traditional elements of community design, in which housing, employment, local commerce, small-scale manufacturing, and recreation were all within easy walking distance.
Urban Land-Use
Urbanized areas cover about 106,386 sq. miles of the U.S., only 3% of the total land. The U.S. is, nevertheless, a highly urbanized country because 82.5% of its population (about 270 million people) lives in cities and towns (World Bank, 2021).
Although patterns of land-use vary among cities in the U.S., the dominant uses are for residential, commercial, industrial, and transportation purposes (Figure 28.2). As such, urban areas are mostly occupied by: single-family homes, duplexes, and apartment buildings; commercial, industrial, and institutional buildings; parking lots and paved roads; and other built structures. Non-paved areas are mostly grassy lawns. All of these urban “habitats” are highly anthropogenic in character – they are, after all, places where large numbers of people live, work, and play. Some urban greenspaces also contain habitat for elements of native biodiversity, as we examine later.
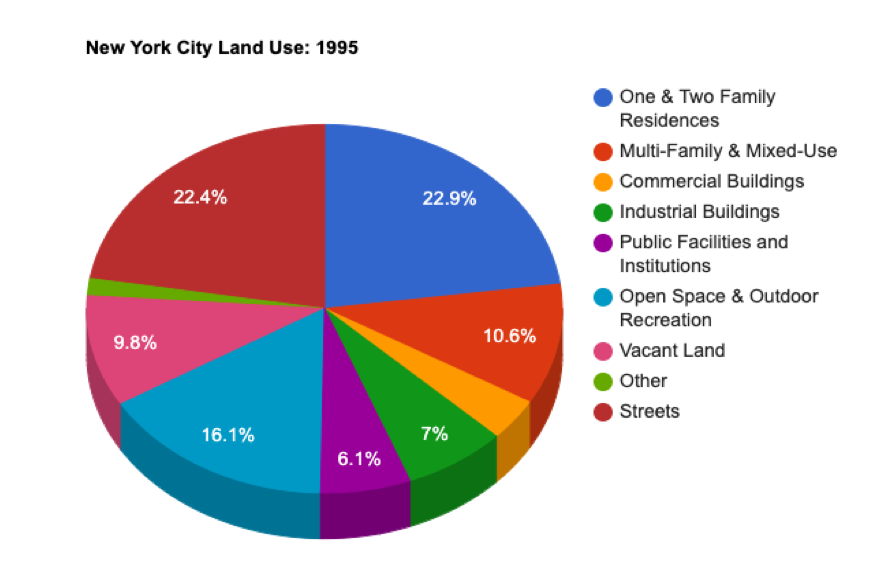
Figure 28.2. Land-Use in New York City. The data show the percentage distribution of land-use in 1995. While this figure is an example, the data for New York City is outdated as it has gone through significant development and land-use changes since 1995. Source: Modified from data from Demographia, 2000, calculated from the City of New York Department of City Planning.
Environmental Issues 28.1. Urban Sprawl
U.S. cities are growing rapidly for two reasons: (1) most immigrants prefer to live in urban areas, and (2) there is immigration from rural districts. Both of these groups are seeking economic opportunities, as well as the cultural and lifestyle benefits of living in large centers. In any event, because of the rapid population growth, urbanized areas are spreading into adjacent rural habitats, a phenomenon known as “urban sprawl”.
All cities and towns in the U.S. are located on land that was formerly occupied by natural ecosystems. However, much of the sprawl that is occurring today involves the conversion of agroecosystems into urbanized areas. When the agricultural land is lost, there is a depletion of the ability of the landscape to provide food.
Urban sprawl is also a threat to natural habitats, particularly in areas that sustain rare ecosystems. For example, the area in South Florida that hosts Biscayne National Park and Everglades National Park are particularly sensitive areas in close proximity to sprawling development. This is habitat to many rare species and endangered natural ecosystems. The expansion of residential areas poses a risk native endangered species such as manatees and the extremely vulnerable Florida panther (Image 28.2). Conservation agencies in government and the private sector, including the Friends of the Everglades, founded by Marjory Stoneman Douglas, are trying to preserve and restore the wetlands and forest.
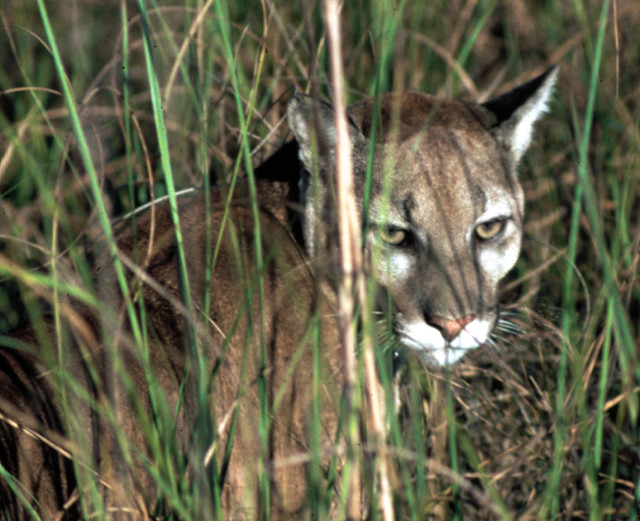
Image 28.2. Florida Panther in the Everglades. Fewer than 100 Florida Panthers are estimated to be left in the U.S. today. Perceived as a threat to livestock, humans and game animals, they were heavily hunted and the species went nearly extinct in the 1950s. Source: “Florida Panther, NPS Photo, 2003” from Everglades National Park, Public Domain.
Urban sprawl can also be a threat to the ability of landscapes to provide key environmental “services,” such as clean water and flood protection. In the case of the Everglades, this is a major concern for habitat protection against further urbanization and sprawl encroachment on the unique wetlands in the South Florida area. Although the region is protected as a National Park, development right up to its boundaries has negative effects on the hydrology throughout the wetlands. The Everglades provide essential ecological services including providing drinking water and irrigation for about one third of Floridians, in doing so, filtering out pollutants and contaminants, as well as serving as natural flood protection for surrounding settlements (Image 28.3). It also serves a valuable recreational purpose and a natural attraction of tourism for visitors within and from outside the state. However, the urban development of nearby cities Miami and Fort Lauderdale, has detracted from the water supply. Additionally, increased visitation and human activity within the park can come at detriment to the wildlife. Floridian farms have also produced phosphorus runoff that has had detrimental effects on the Everglades ecosystem.
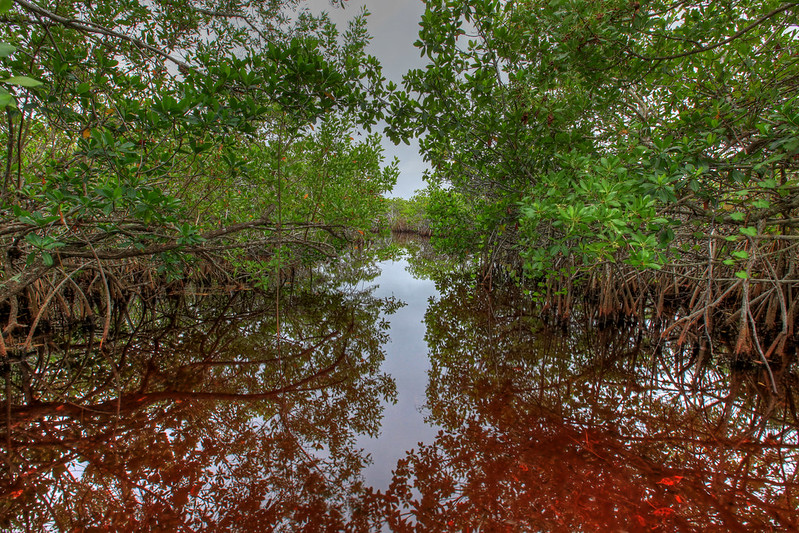
Image 28.3. Mangroves in Everglades National Park. Mangroves serve as nursery habitats for a variety of marine species and also help in flood control. The Everglades National Park protects the largest contiguous stand of mangrove forests in the western hemisphere. Source: “Mangrove water, NPSphoto, G.Gardner” by Everglades National Park, Public Domain.
However, the protection of these natural areas has not had unilateral support and the boundaries of preservation continue to be an ongoing battle within the state government. Developers are insistent on reclaiming rights to build on land that crosses the protective boundary, as well as continuing to purchase land bordering the Park that is not zoned for development. They do so with the intention of pressuring politicians to renegotiate these terms so they can develop, but this was and continues to be met with stark public and activist opposition (Lilly, 2012).
Urban Transportation
All urban areas have complex systems for moving people and goods. The physical infrastructure for transportation includes roads, parking lots, railroads, subways, airports, and water routes, plus the many kinds of vehicles that operate on these corridors. Public transit is still an important means of transportation in cities, although its growth is moderate and not nearly as impressive as that of automobile use (Figure 28.3).
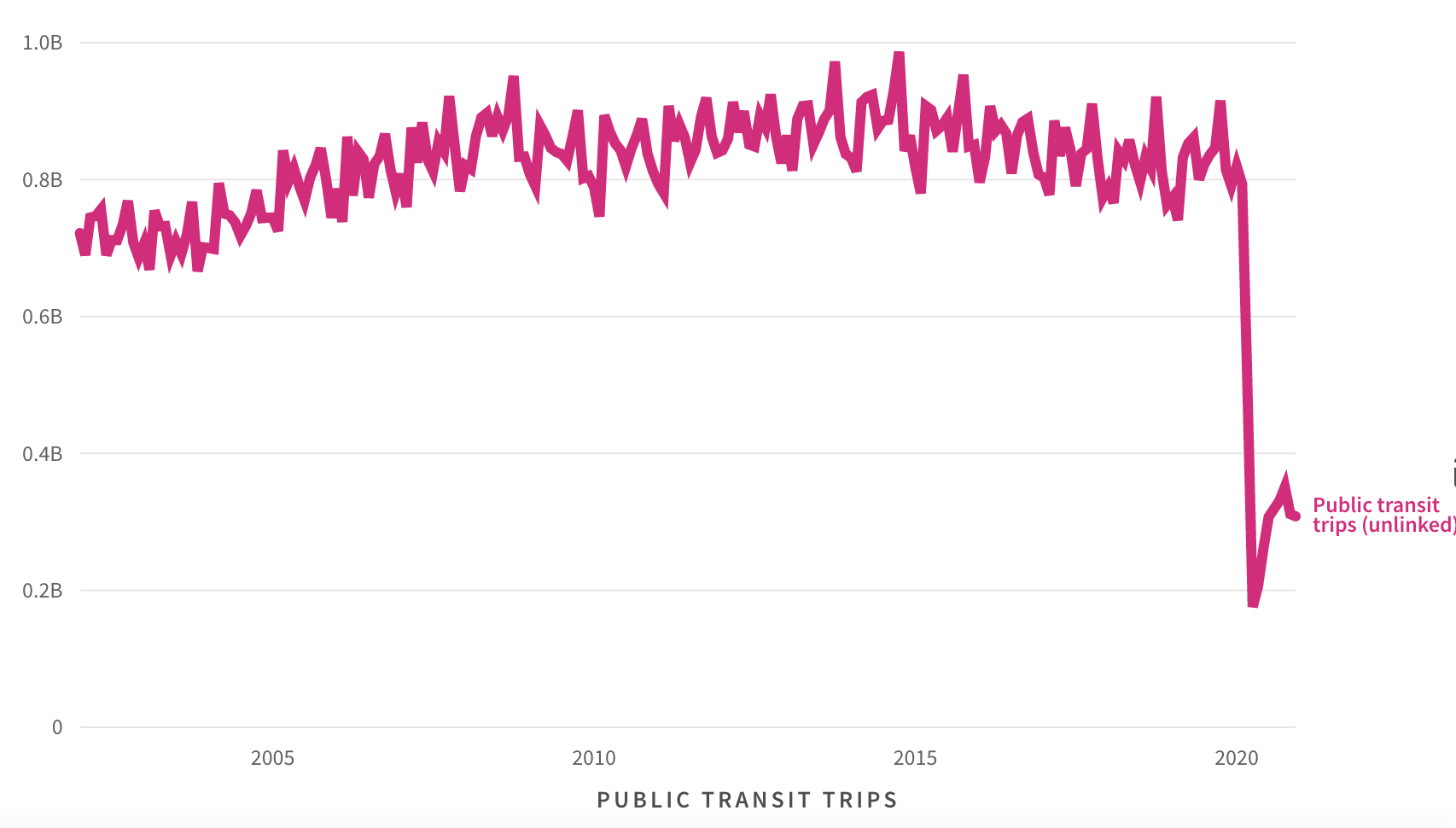
Figure 28.3. Changes in Public Transit Ridership in the United States. The data show the trends in the use of unlinked public transit trips. The drastic drop in the data series in 2020 reflects the impact of the Covid-19 pandemic with stay-at-home orders issued across the U.S. Source: USAFacts under CC BY 4.0, based on data compiled by the Department of Transportation.
Most urban transportation involves the use of motorized vehicles. U.S. transportation infrastructure is overwhelmingly focused around automobiles and motorized vehicles rather than trains or light rail. On average, at least 85% of commuters travelled by private vehicle (car or light truck), and only 5%, by bus; or rapid transit and rail; about 5% work from home; almost 3% walked; and less then 2% commuted by other means such as bicycles (Figure 28.4). In general, larger cities have a better developed infrastructure for public transit. For example, in 2019 in Metropolitan New York, more than 7 million people used public transit on an average weekday, with more than 75% of which is on the MTA subway system and the remaining on New York City Transit buses (MTA, 2020).
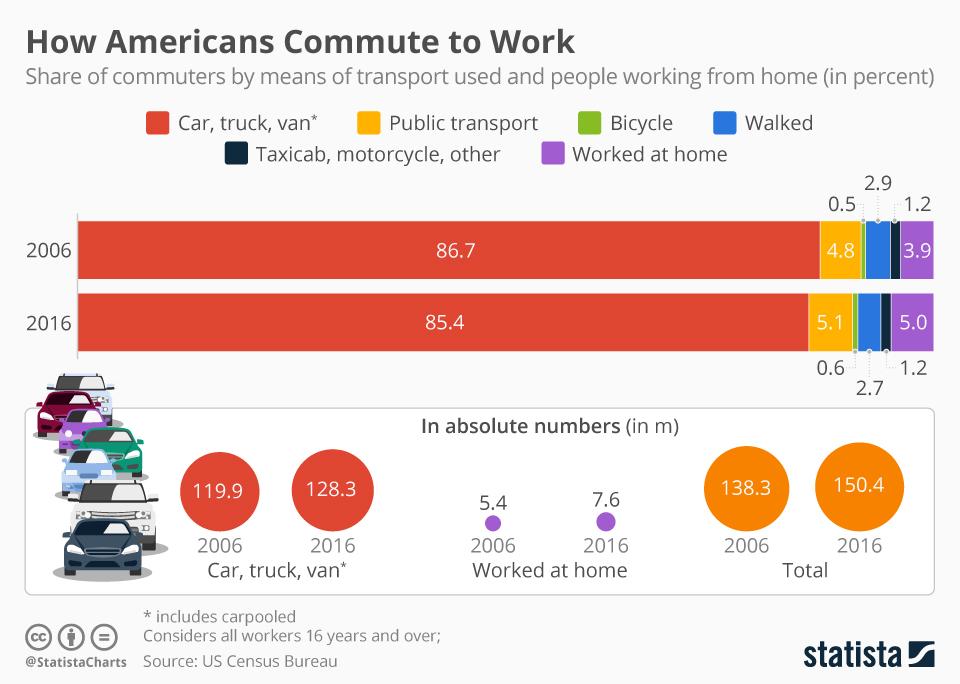
Figure 28.4. Means of Transportation for Commuting to Work Data is a comparison between 2006 and 2016. The top comparison and are percentages of the total commuters while the bottom depicts the raw number in millions. Source: Statista, 2017 licensed under CC BY, compiled from data from the U.S. Census.
These general patterns are also shown by the costs of transportation (Figure 28.5). The Federal government invests significantly more money into the highway system to maintain the existing automobile-dependent infrastructure compared to other vital services such as water utilities. Typically, half of federal transportation spending goes toward maintaining highways with about 20% going toward air travel (USA Facts, 2021). Therefore much less is invested towards improving mass transit or expanding pedestrian and bicycle infrastructure, even though these are the most energy-efficient means of transportation in urban areas (see In Detail 28.1).
Figure 28.5. Federal Expenditures of Transportation in the United States in 2017 in U.S. dollars. Source: USAFacts, 2021 licensed under CC BY. Data from the Congressional Budget Office.
Although owning a car or truck is widely viewed as a desirable aspect of our lifestyle, the reliance on personal motor vehicles seriously affects environmental quality in cities and towns (Image 28.4). Compared with other means of urban transportation, personal motor vehicles emit more air pollution, require more physical infrastructure (such as roads and parking space), use more material and energy resources, and are more costly to own and operate. Significant safety hazards are also associated with the use of personal motor vehicles. For example, 36,560 people died in motor vehicle accidents in 2018 in the U.S., equivalent to 1.3% of all deaths (NHTSA, 2019).
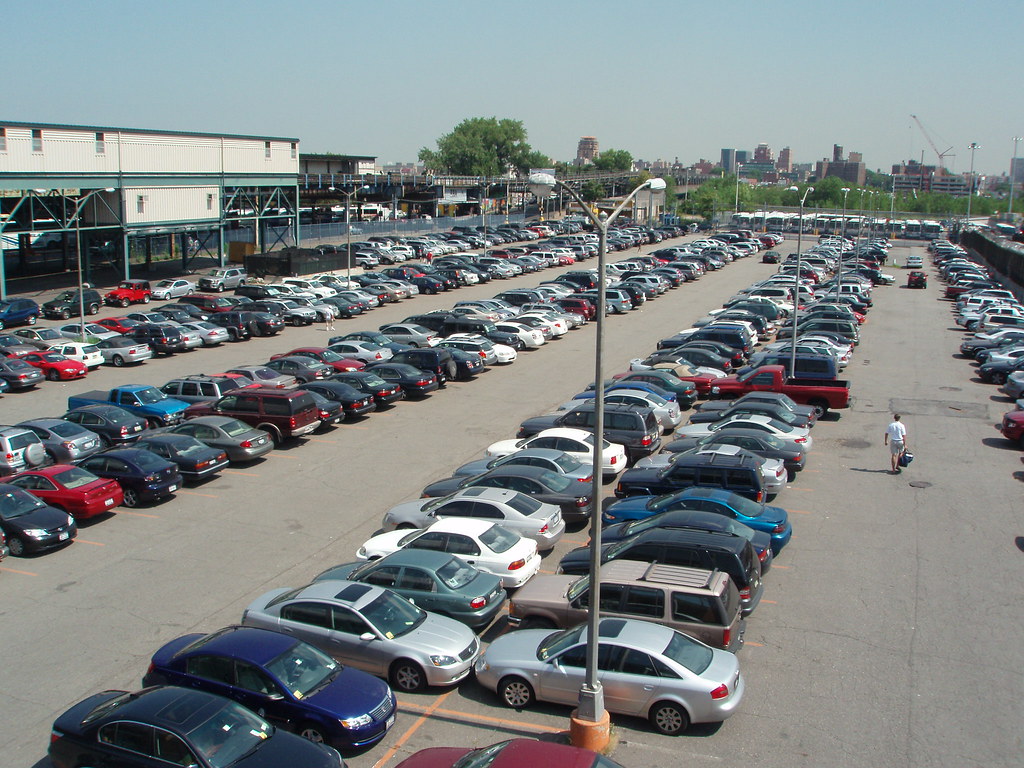
Image 28.4. A large amount of space is allocated to cars and other vehicles in cities, particularly roads and parking areas. If intelligent life forms on a voyage of discovery were to hover in their spacecraft over a U.S. city, they might initially conclude that the dominant form of life is the automobile. Source: “US Open Parking Lot” by Alex92287 is licensed under CC BY 2.0.
In Detail 28.1. The Bicycle: A Green Machine
Invented in the late nineteenth century, the bicycle rapidly became recognized as an efficient, safe, and fun way to get around. Even today, with all of the advances in technologies for powered transportation, the bicycle is still the most efficient means of transporting people on land. This fact is illustrated by comparing the energy typically used to transport a passenger using various technologies (Holcomb, 1987):
- Automobile (1 occupant) 1163 cal/km
- Automobile (2 occupants) 581
- Transit bus 575
- Transit rail 553
- Walking 63
- Bicycling 22
Unlike motorized transportation, riding a bicycle does not emit air pollutants such as NOx, SO2, hydrocarbons, or particulates. Moreover, bicycle manufacturing requires far less material and energy resources, and less infrastructure is needed to support these vehicles in terms of space for roads and parking. Therefore, bicycles represent an environmentally soft (or “green”) alternative to motorized vehicles for personal transportation. There are more than 1-billion million bicycles in the world, about half of them in China. In spite of this number, only about 1% of all trips are made using a bicycle, which suggests that many bicycles are not well used for transportation purposes. Great environmental benefits would result if more Americans used bicycles instead of motor vehicles as a means of personal transportation. These benefits include less air pollution, smaller costs of transportation infrastructure, and less use of energy and material resources. Personal benefits include the convenience of bicycle travel and improved health from frequent exercise.
However, bicycle riding can be dangerous, mostly because of the risk of collisions with motor vehicles. Even though bicycles only account for 1% of trips across all modes of transportation, U.S. bicyclists die double the rate of vehicle occupants. However bicycle fatalities have decreased 44% from 1975 to 2012 (Vargo et al., 2015). Safer bicycle use requires separate lanes along busy roadways, improved awareness by automobile and truck drivers of the need to share the road with bicycle riders (and vice versa), and increased use of safety equipment by bicyclists, especially helmets and lights and reflective devices at night. The use of bicycles in cities can be encouraged in the following ways:
- By developing a network of cycleways (separate paths for bicycles), bike lanes, and paved shoulders on major roads
- By providing secure parking facilities at workplaces and public-transit stations (the latter is known as “bike and ride”)
- By having a fleet of bicycles for free use or inexpensive rental from a network of pick-up centers (this system, first used in several European cities, is being tried on a smaller scale in North America)
- By educating people about the advantages of bicycle transport and safety issues
Other ways to increase bicycle use would be much more difficult to implement. For example, urban-planning options could encourage people to live closer to their workplaces, giving them shorter commutes. In addition, legislation could ensure that people using motorized transport pay the full costs of their choice of transportation by withdrawing subsidies for the construction of roads, bridges, parking lots, and other infrastructure and by applying realistic taxes (such as user fees) to offset the costs of associated air pollution and resource depletion.
Urban Pollution
Urban pollution includes elevated concentrations of chemicals and particulates, increased noise and heat, and impaired aesthetics. We briefly examine each of these topics in the following sections.
Chemical Pollution
Urban environments commonly have higher concentrations of various chemicals than typically occur in rural places. As well, a wider range of chemicals is present, including many synthetic ones. Often, chemical pollution of the urban environment is intense enough to damage the health of people, animals, and vegetation.
Soil in urban areas may become polluted by a wide range of substances, including petroleum products, halogenated hydrocarbons (such as PCBs), and metals. This is illustrated by the following examples:
- Hydrocarbon spills: Liquid hydrocarbons, such as gasoline, fuel oil, paints, and solvents (such as those used in dry-cleaning) are stored in many places. This includes underground tanks at gasoline stations and fuel-oil tanks in homes and at commercial, industrial, and institutional buildings. Smaller amounts of fuels are contained in all cars, trucks, and other vehicles. Spills of some kinds of liquid fuels, such as gasoline, can be extremely hazardous because of the risk of fire or explosion. In addition, if liquid hydrocarbons are spilled onto soil, they quickly penetrate and cause local pollution of the groundwater, which may then spread widely as a plume of tainted water. This can ruin the usefulness of an aquifer as drinking water: as little as 1 ppm of hydrocarbons can result in discernible tainting and even smaller concentrations of some chemicals are considered a health risk. It is difficult or impossible to contain a hydrocarbon spill once it reaches groundwater or to fully rehabilitate a polluted aquifer. It is much easier to avoid hydrocarbon spills than to deal with the environmental damage they cause.
- Metals: Urban soil can become polluted by metals in many ways. For example, people who live near metal-recycling factories may be exposed to pollution through dustfall from the atmosphere. In one case, severe pollution was found in a downtown Toronto residential neighborhood near a car-battery recycling factory. Lead in surface soil near the factory exceeded several thousand ppm (one sample had >5% lead), and some residents had elevated lead in their blood and hair. This astonishing land-use conflict occurred because of earlier, inappropriate land-use zoning, when less attention was placed on environmental concerns. However, the battery-recycling factory still remains in the residential neighborhood, although it operates much more cleanly than in the past. Lead pollution of urban soil has also been caused by residues of old paint, which often had very high concentrations of lead-containing pigments (commonly reaching 38% in the dried residue), especially in white and red colors. (Lead is now mostly replaced in household paints by titanium and other less-toxic metals). Soil close to busy highways is also affected by leaded gasoline (this problem has dissipated since the 1990 ban on leaded gasoline; see Chapter 22)
Eutrophication is another kind of urban pollution. Lakes and rivers in urban areas often have an enriched nutrient supply, which results in increased productivity and eutrophication. The nutrient inputs originate from fertilizer use in horticulture, leaching from septic fields, and sometimes the dumping of sewage. Urban waterbodies are also commonly polluted with coliform bacteria and other intestinal pathogens and parasites that originate with sewage and surface runoff contaminated by pet and bird feces. Lakes and rivers may also be affected by spilled fuel, metals, garbage dumping, and sedimentation by soil eroded during construction activities. Any of these influences on urban lakes may render them less suitable or even unfit as a source of drinking water or for recreational purposes. Eutrophication and sedimentation also cause significant ecological damage in many urban waterbodies.
Urban air pollution can also be severe, causing life-threatening health problems for many people, particularly the old or very young and those with respiratory diseases. Severe pollution by sulfur dioxide and particulates was once common in North American and European cities, when coal was burned as an industrial and residential fuel. This problem has, however, been greatly alleviated since the early 1960s because of clean-air regulations (Chapter 20). However, this kind of reducing smog is still severe and even worsening in many rapidly developing countries, where a lower priority is given to enforcing clean-air laws.
In spite of great improvements since the 1960s, cities in the U.S. and other developed countries continue to be affected by significant levels of air pollution (Chapter 20). The following are particularly important:
- SO2 and NOx gases: Although so-called reducing smog is much less of a problem than before, urban atmospheres still have higher concentrations of SO2 and NOx than rural areas. The dry deposition of these gases damages marble, limestone, sandstone, and other acid-sensitive building materials and statues, and it contributes to the acidification of aquatic habitats (Chapter 23). In addition, these gases may be directly toxic to sensitive urban plants and lichens.
- Suspended particulates: Largely originating from emissions from vehicles, furnaces, and other combustion sources, suspended particulates are mostly fine aerosols of ammonium sulfate, ammonium nitrate, and sooty organics. At high concentrations they form a haze that interferes with visibility and is a hazard to people with respiratory problems. In general, air pollution by particulates has decreased markedly during the past several decades, mainly because of better emission controls on power plants, other industrial sources, and vehicles, and because of widespread switching to natural gas as a fuel.
- Oxidants: Cities with a sunny climate and a large number of motor vehicles often develop a photochemical smog during the day, characterized by high concentrations of ozone, peroxy acetyl nitrate (PAN), NOx, and volatile organic compounds (Chapter 20). The ozone component is responsible for most of the oxidant damage because it is toxic to many plants, irritates people’s eyes and respiratory tracts, and degrades many materials and pigments.
Urban Climate
The climate of urban regions is different from that of nearby rural areas. Compared with rural areas, cities typically exhibit the following characteristics:
- Are warmer by 3-6°C (37ºF – 43ºF)
- Have 5-10% more cloud cover
- Receive about 20% less solar radiation
- Have 20-30% lower wind speeds (although wind-tunneling may increase windspeeds near large buildings)
- Have 5-15% lower relative humidity
- Receive 5-15% more precipitation
The best known of the climatic differences, called the “heat-island” effect, refers to the warmer temperature of cities, which occurs because of the following influences on the urban energy budget:
- An emission of large amounts of heat (thermal energy) from buildings and machines (including motor vehicles)
- Interference by buildings with the dispersal of warmed air by wind
- The absorption of solar radiation by dark surfaces (especially asphaltic roads and parking lots), followed by the re-radiation of long-wave infrared
- A relative lack of plant foliage in urban areas (the atmosphere is cooled by the evaporation of water from foliage, also known as transpiration)
Thermal Pollution
Thermal pollution occurs when an increase in environmental temperature is sufficient to result in ecological damage. This may be due to the direct discharge of heat into the environment, often from a point source into an aquatic ecosystem. As the water becomes warmer, the respiration of poikilothermic (cold-blooded) organisms increases, about doubling in rate for every 10°C (or 50ºF) increase in temperature. At the same time, the amount of dissolved oxygen in the water decreases (because the solubility of gases is less in warm water). The increased metabolism and decreased oxygen cause physiological stress to aquatic organisms. Associated ecological problems include changes in the communities of fish, invertebrates, aquatic plants, and algae. Extreme thermal pollution can result in anoxic water, accelerated eutrophication, and fish kills. Thermal pollution can reduce the potential for recreational, industrial, and drinking-water uses of a waterbody.
Thermal pollution is associated with discharges of warmed water from fossil-fueled or nuclear-power plants or other large industrial facilities. These places often use a local waterbody as a source of cooling water. The warmed water is then returned to the environment to dissipate its increased heat content. Because power plants are typically 30-40% efficient in converting the energy content of their fuel into electricity, the other 60-70% of that energy must be dissipated. A power plant employs heat exchangers to warm the cooling water, which is then released into the environment.
However, some receiving waterbodies may be too small to absorb all of the heat received without becoming excessively warmed (for example, by more than about 5°C or 41ºF) and suffering ecological damage. In such cases, some of the heat may be dissipated into the atmosphere using special facilities, such as a constructed, shallow cooling pond, in which surface evaporation cools the water before its release into the natural environment. Alternatives include a “wet” cooling tower, in which the water is cooled by spraying it into the air, or a “dry” cooling tower, which uses a system of heat exchangers to transfer heat to the atmosphere (these options are uncommon because of their high costs of construction and operation). Better yet, some of the “waste” heat may be used to warm nearby buildings, or in commercial greenhouses to grow vegetable crops in winter, or in an aquaculture facility to grow fish (this is more commonly done in Europe).
Noise Pollution
Urban areas are typically much noisier than rural ones. Most of the noise comes from the operation of various kinds of machines that are abundant in urban places, such as air conditioners, lawnmowers, automobiles, heavy trucks, and air compressors. Loud music may also be important. Noise pollution begins when the level of ambient sound becomes distracting to the normal activities of people, for example, by making it difficult to understand a conversation or to have a restful sleep.
Noise intensity (also called sound pressure or loudness) is measured in units of decibels (dB). An increase of 10 dB is approximately equivalent to a doubling of the loudness. When the distance from a point source is doubled, the noise level decreases by about 6 dB. However, when the distance from a linear source of noise (such as a busy highway) is doubled, the sound level decreases by only about 3 dB.
At a high intensity, noise pollution can cause a progressive and enduring hearing impairment, which begins with a decreased ability to perceive higher-pitched sound. Under prolonged exposure, the hearing loss may progress through much of the auditory range. Hearing loss is caused by both the duration and intensity of exposure. For example, an occupational exposure to 115 dB may be permitted for no longer than 15 minutes, while exposure to 100 dB may be allowed for two hours and 90 dB for up to eight hours. Noise becomes painful at about 140 dB, although prolonged exposure to levels above 80-90 dB can result in long-term hearing loss. The noise levels typically associated with various sources are shown in Figure 28.6.
People may be exposed to excessive noise in various ways. Non-voluntary exposures occur in the ambient environment, such as near a road with heavy traffic. Occupational exposures occur in noisy factories or in hangars where jet engines are serviced. Voluntary exposures to excessive noise are also common, such as when people attend thunderous rock concerts or use headphones to listen to loud music. It is well known that permanent hearing loss is common among rock musicians as well as people who regularly listen to loud music at concerts, clubs, or using headphones. Frequent target-shooting with firearms can also result in long-term hearing loss unless effective ear protection is used.
Various levels of government in the U.S. have set criteria for permissible exposures to noise. The New York City Noise Code lists regulations and guidance for a variety of noise sources in the city including refuse collection vehicles, construction, and bars and restaurants. For example, the NYC Noise Code states that music from commercial establishments cannot exceed sound levels of 42 dB as measured from inside nearby residences (NYC DEP, 2018). Different actions can reduce the noise intensity in occupational and urban environments such as vehicles and other machines having noise-absorbing devices (mufflers) and workers in particular work environments being advised or required to wear hearing protection.
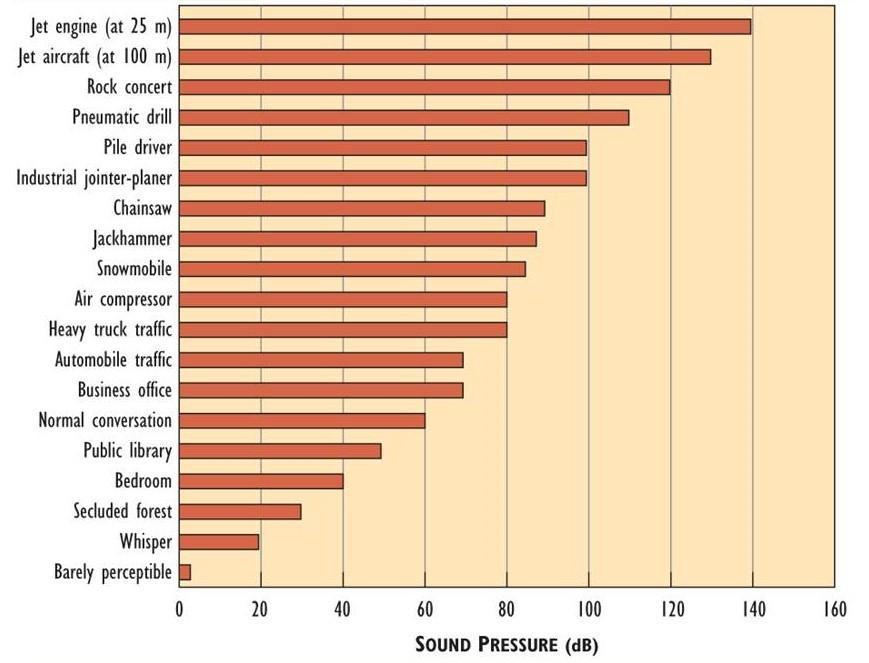
Figure 28.6. Sound Pressure Levels Associated with Various Sources of Noise. Source: Data from Timerson (1999).
Aesthetic Pollution
Aesthetic pollution is substantially a matter of cultural values. It commonly involves visual images that are displeasing to many (but not necessarily all) people. As such, the criteria for aesthetic pollution cannot be precisely defined. For example, many people might find the following to be objectionable:
- A neighborhood of dirty run-down buildings
- An area cluttered with garbage
- A street with a viewscape blocked with gaudy billboards, neon advertising, and other promotions
- Overhead utility wires
- Spaghetti-like networks of roads, overpasses, and underpasses crowded with grid-locked vehicles
- Paved open spaces without trees, shrubs, or flowers
There is, however, no broad consensus about what constitutes aesthetic pollution – some people might find that these scenes have aesthetic merit and may even seek them out. Aesthetic pollution is not generally considered to be as important a problem as chemical, noise, or thermal pollution. Nevertheless, urban planners, neighborhood activists, and other concerned people do try to reduce the aesthetic pollution of cities and towns. For example, many urban areas have bylaws that require property owners to keep their property clean and in good repair. Some places require that utility cables be buried, or they ban certain kinds of advertising. (For example, billboards are banned in the state of Vermont.) Reducing aesthetic pollution in urban areas makes them nicer places in which to live and work.
Waste Management
Any discarded materials can be viewed as waste, but there are a number of categories:
- Solid wastes are extremely variable in composition and include discarded food, leaves and lawn clippings, newspapers and other papers, glass and plastic bottles, cans, disposable diapers, construction debris, industrial chemicals, old cars, and disused furniture.
- Liquid wastes include sewage and discarded industrial and household fluids.
- Gaseous wastes include products of combustion or industrial reactions.
- Hazardous wastes are flammable, corrosive, explosive, toxic (also called toxic waste), or otherwise dangerous, and they should either be treated before disposal or discarded into a specially designed, secure landfill to environmental damage.
People have always produced wastes of many kinds. However, the amount and complexity of discarded materials have increased enormously as a result of industrial and technological development, coupled with the growth of population and consumerism (Image 28.5). Activities in urban areas produce huge amounts of waste. Waste management is the handling of discarded materials, using various methods:
- Dumping is the long-term disposal of disused material. The disposal of solid wastes by dumping usually occurs into a sanitary landfill (see below). Liquid wastes are most commonly discarded into a nearby lake or river, with or without treatment to reduce the amounts of organic matter, toxins such as metals and hydrocarbons, and pathogens. Gaseous wastes are usually dumped into the atmosphere, although the amounts of damaging gases (such as SO2) and particulates may be reduced by pollution-control technologies.
- Incineration is the combustion of solid wastes to reduce the amount of organic material. Small-scale incineration may involve open burning. However, incineration in urban areas and industrial plants is conducted in specially engineered facilities designed to burn efficiently, while controlling the emissions of pollutants to the atmosphere. The residual material, consisting of ash, metals, glass, and other non-combustibles, is usually disposed of in a secure landfill. Overall, incinerators reduce the volume of waste by 70-90%, depending on the initial organic content. If the heat produced is used to generate electricity or industrial steam, the incinerator is known as a waste-to-energy facility.
- Recycling involves the processing of discarded materials into useful products. For example, aluminum pop cans can be collected and re-manufactured into new containers or other products. Recycling is an extremely attractive waste-management option for three major reasons: (1) it reduces the total amount of waste, (2) it recovers valuable commodities from discarded materials, and (3) it helps to conserve non-renewable resources (such as metals and fossil fuels) and some renewable ones (such as trees used to manufacture paper). Easily recyclable materials include glass, all metals, most plastics, and almost all kinds of paper. In fact, these discarded materials should be regarded as “resources” rather than “wastes.”
- Composting is a type of recycling in which discarded organic materials are allowed to partially decay under warm, moist, oxygen-rich conditions. Backyard composting is done in simple bins or piles. To enhance the availability of oxygen, advanced commercial systems turn the material occasionally or force air through matter contained in a vessel. All food wastes, lawn clippings, leaves, paper, sawdust, sewage sludge, and other organic discards can be composted. The material produced, known as compost, is rich in humified organic matter and is extremely useful in enhancing the tilth and fertility of garden and agricultural soil (see Chapters 12 and 13).
- Re-use involves finding another use for discarded materials, usually with relatively little modification. Re-use is an attractive waste-management option for the same reasons noted for recycling, but it is even more effective at conserving resources because little effort is put into re-manufacturing (other than repairs, if necessary). Re-use is a common practice with discarded furniture, appliances, books, tools, clothes, and other consumer products. Increasingly, institutions and industries are finding ways to re-use paper, cardboard boxes, other containers, wooden pallets, and other disused materials. Often, re-using networks are organized among industries, universities, hospitals, and other institutions, because “waste” materials produced by one partner may be a “resource” for another.
- Waste reduction and prevention are not, strictly speaking, waste-management methods. Rather, they are intended to reduce the amounts of waste that must be handled by the above methods. Waste reduction and prevention include choices by consumers to buy products that are not excessively packaged or that are sold in reusable, returnable containers. People can also choose to buy less –to have fewer shoes and items of clothing, to own fewer (or no) automobiles, and to adopt other elements of a less consumerist lifestyle. Industries also have many options to reduce or prevent their production of wastes. For example, wood-processing industries once routinely incinerated or land-filled waste tree bark and sawdust, but today they routinely use them to manufacture pulp or as a source of energy. In fact, some pulp mills now satisfy most of their needs for raw fiber by using sawdust and trimmings from nearby sawmills.
The various ways of managing waste materials differ greatly in their environmental impacts. In general, environmental problems associated with the generation and disposal of wastes are lessened by adopting any of the following “R’s” into our individual and corporate lifestyles: refuse (waste prevention), reduce, reuse, and recycle.
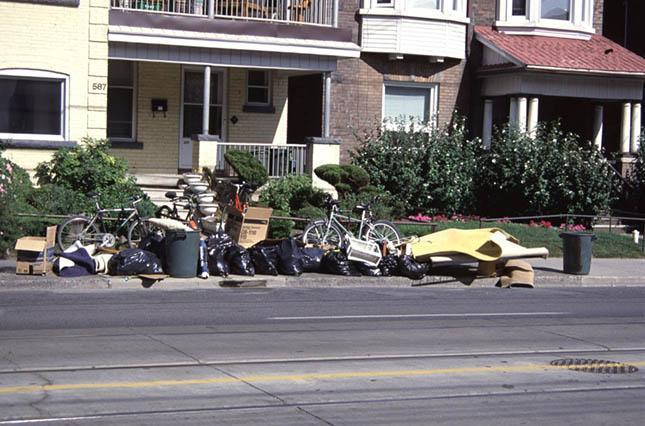
Image 28.5. Solid Waste in a Residential Neighborhood. Solid-waste management is an important function carried out by municipal governments. This image shows a pile of discarded material placed at the curbside for collection. Much of this material could have been recycled, but instead it will be land-filled. Source: B. Freedman.
Municipal Solid Waste
Municipal solid waste (MSW) is generated by households, businesses, and institutions such as schools and hospitals. The components of MSW are extremely diverse, but they do not include sewage sludge (discussed in the following section) or waste generated by heavy industry. Some MSW is disposed in open dumps that are usually located in a relatively out-of-the-way natural basin, such as a lake, wetland, or other low area, which is gradually in-filled with waste. Many environmental damages are associated with open dumps, including the pollution of groundwater and surface water by toxic leachate, foul smells, smoke from open burns used to decrease the volume of garbage, populations of pest animals, and terrible aesthetics.
In the 1920s, engineers began to design sanitary landfills, where MSW is dumped, compacted by heavy machines (such as bulldozers), and covered with about 10 cm of clean dirt at the end of each day. This reduces odors and populations of rodents, gulls, insects, and other pests. Sanitary landfills became common in developed countries during the 1940s, and advanced variations are now used by U.S. municipalities. Modern sanitary landfills include the following elements in their construction:
- One or several impervious linings of clay, plastic, or concrete that prevent the downward leaching of water with high concentrations of ammonium, metals, and other toxic chemicals
- A cutoff wall of concrete or another impervious material around the edge of the landfill to prevent the sideways migration of polluted water
- A system of pipes and gravel drains to collect leachate for treatment before the water is released into the environment
- A system of piping to collect methane, a greenhouse gas produced by anaerobic decomposition of organic matter in the landfill, which can be burned to produce electricity or heat
In spite of these advanced design elements, residual problems of groundwater and surface water contamination and methane emissions to the atmosphere may still occur.
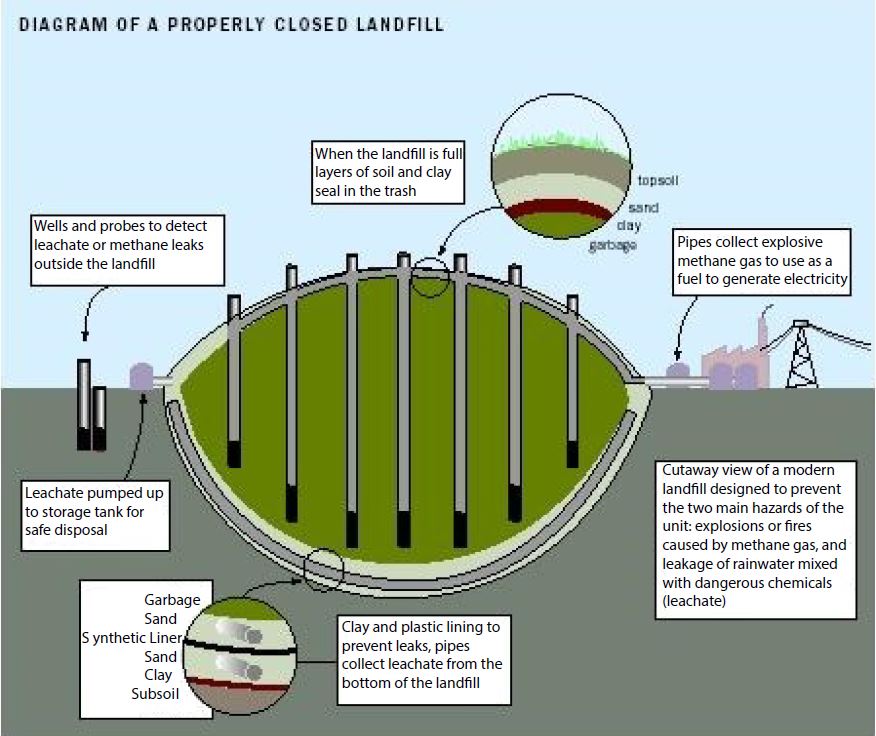
Figure 28.7. Diagram of a Properly Closed Landfill. This cross-section diagram depicts a properly sealed landfill that captures waste methane from anaerobic decomposition. Source: EPA.
Another way of disposing of MSW is to incinerate it. During the nineteenth century, some cities burned much of their organic garbage using crude facilities known as cremators or cone-shaped teepee-burners. However, these caused intense local pollution and were replaced by the land-filling of MSW. Beginning in the 1930s, some cities built more-efficient burning facilities called incinerators, but these were also dirty and most were shut down by the late 1960s. However, beginning in the 1970s, much cleaner incinerators were built, known as waste-to-energy or resource recovery facilities. These incinerators burn organic MSW efficiently and have technology installed to reduce the emissions of particulates and other air pollutants. However, the ash produced is a toxic waste that must be disposed of in a secure landfill.
All municipalities need facilities for handling their solid wastes. However, public attitudes play a crucial role in any waste-management decisions. Any new proposal to develop a sanitary landfill, incinerator, recycling plant, or other MSW-related facility is sure to be opposed by people living near the proposed site. This phenomenon is referred to as NIMBY, an acronym for not in my backyard. If local opposition becomes highly vocal and widespread, municipal bureaucrats and politicians often will not approve a proposal, which creates an important problem for those who are attempting to deal with waste-management issues. It also strongly motivates engineers to design landfills and incinerators to operate as cleanly as possible, while increasing the pressure for society to act to reduce and recycle discarded materials more efficiently than in the past.
In 2018, Americans produced over 292.4-million tons of MSW (Figure 28.8). Much of this dumped MSW could be diverted since the majority consists of recyclables (paper, metals, glass, plastics) and organic waste (yard trimmings food waste), indicating a large potential for composting. Note, however, that these figures can vary widely across the U.S., depending on the size and density of the urban population (diversion programs are more economic in larger centers) and on the priority placed by municipal and provincial/territorial governments on advanced (but expensive) options for the management of solid wastes. Increasingly, governments are requiring or encouraging options that decrease the amount of solid waste that must be handled by land-filling or incineration.
Figure 28.8. Total Municipal Solid Waste by Material in the U.S. for 2018. Source: EPA.
Municipal Wastewater Treatment
Issues associated with municipal wastewater include its amount and composition, ways of treating the material, and ecological effects of its disposal. First, we should distinguish among major kinds of wastewater.
- Sewage is wastewater that contains the fecal matter of humans and other animals, plus food waste from kitchens and commercial food processing. In urban areas, sewage is collected using a complex system of underground pipes called sanitary sewers, and transported to a central place for treatment and/or disposal.
- Industrial wastewater may contain many kinds of liquids, including toxic and hazardous wastes. As was previously noted, toxic waste is poisonous, while hazardous waste may be explosive, flammable, or dangerous for other reasons. Many urban areas have separate systems to collect and treat toxic and hazardous industrial wastewater.
- Stormwater is the runoff of rainfall and snowmelt. In municipal areas, it is typically collected using surface drains that feed into a combined sewage-stormflow system, or using a more advanced drainage system that keeps these wastewaters separate. Stormwater is not as grossly polluted as sewage or industrial wastewater, but it does contain significant amounts of fecal material (from pets and wild animals), metals, waste motor oil, road salt, and other substances.
Sewage Treatment
In most places, the principal objective of sewage treatment is to reduce the amounts of pathogenic microbes and oxygen-consuming organic matter that are disposed of into receiving waterbodies. In places where surface waters are vulnerable to eutrophication, sewage may also be treated to reduce the amounts of nutrients, especially phosphorus and nitrogen (see Chapter 24; Image 28.6).
All towns and cities have networks of underground pipes to collect the sewage effluent from homes, businesses, institutions, and factories. (However, low-density residential areas may have septic systems installed at individual homes.) Some municipalities have separate collection systems for sewage and stormwater. Eventually, all of the wastewater is discharged to the environment, usually into a nearby lake, river, or ocean. To avoid environmental damage, the wastewater should be treated to reduce its pollutant load before it is discharged. However, some towns and cities still dump raw sewage. Most of these municipalities are located beside an ocean and rely on the local, well-flushed marine ecosystem to dilute and biodegrade organic pollutants and pathogens in the sewage. Because inland waters such as lakes and rivers have a much smaller capacity for diluting and biodegrading sewage wastes, municipalities located beside these waters treat their sewage. (See Chapter 24 for a description of various sewage-treatment systems.)
More than 75% of Americans are serviced by centralized wastewater collection and treatment systems, the rest use septic tanks or other private onsite systems. In the year 2000, about 208 million people in the U.S. were serviced by a centralized wastewater collection and treatment plant. Combined sewage collection systems that were primarily installed up to the first half of the 20th century combine stormwater drainage and sewage collection, resulting in raw discharge in the case of stormwater overflows. These systems are no longer sanctioned to be installed and instead only Sanitary Sewage Collection, which separate storm runoff and sewage collection, are implemented to lessen the discharge of untreated or poorly treated sewage into the environment (EPA, 2004). Environmental damages associated with the discharge of untreated or poorly treated sewage include the following:
- The pollution of receiving waters with human fecal pathogens, such as coliform bacteria and viruses, which renders the area unfit for swimming and for use as drinking water
- Ecological damage caused by the deoxygenation of water and sediment by the decomposition of organic wastes, resulting in the deaths of many organisms and the development of foul odors
- Stimulation of algal blooms through excessive nutrients from sewage effluent
- Contamination of the environment with persistent, potentially toxic chemicals, such as metals and organochlorines
- Aesthetic damage associated with the presence of sewage waste
These environmental damages, which can be severe, are largely avoided if municipalities invest in facilities to treat their sewage.
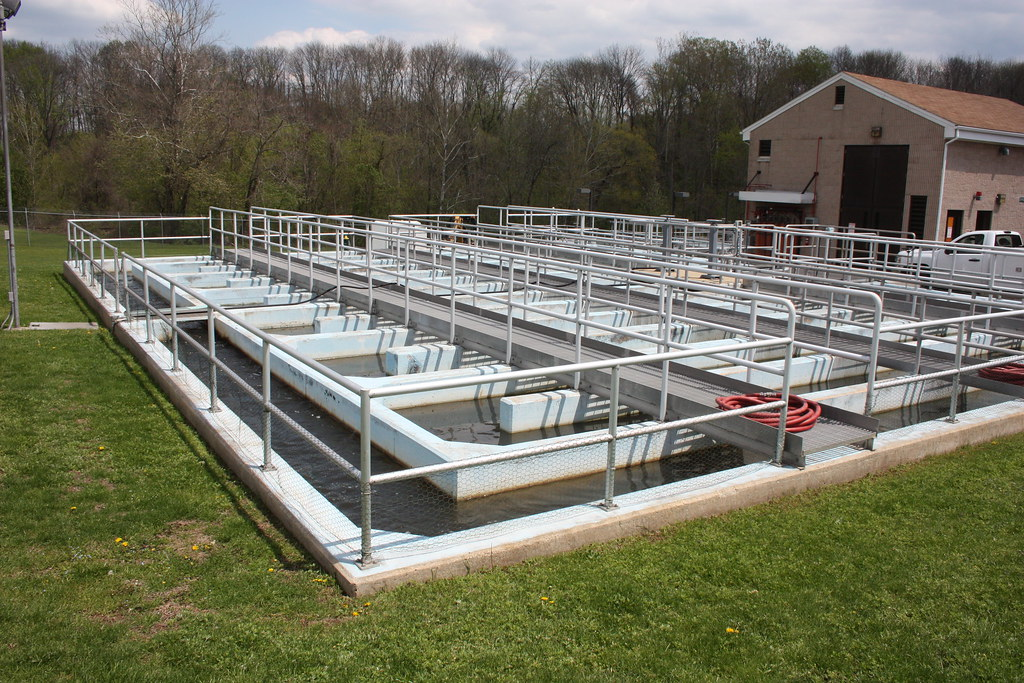
Image 28.6. Trout Run Sewage Treatment Plant. The Trout Run Water Pollution Control Center is located in the township of Upper Merion, Montgomery County, Pennsylvania. This sewage-treatment complex serves more than 8,500 private residences and about 800 commercial establishments. The treated effluent goes into the Schuylkill River, a relatively small waterbody. Source: “Trout Run Sewage Treatment Plant II” by Montgomery County Planning Commission is licensed under CC BY-SA 2.0.
Urban Biodiversity
Urban areas are highly impoverished in terms of the amount and quality of habitat available to support plants, animals, and microorganisms. Nevertheless, many wild organisms do occur – even parking lots, sidewalks, and industrial areas, which from an ecological perspective are extremely degraded habitats, do manage to support some biodiversity.
Still, some urban places are relatively natural in character and are maintained in this condition as parks. Despite the U.S.’s well-known and visited National Park System, some cities have impressive natural areas of their own. Some prominent examples of “natural-area parks” include Forest Park in Portland, Oregon (Image 28.7) and the Great Trinity Forest in Dallas, Texas. A respective 5,000 and 6,000 acres make these urban forests the large natural areas within the city limits of a metropolitan area. These greenspaces are remnants of natural habitat that have survived the urbanization process, and they contain ecological communities that are mostly dominated by native species.
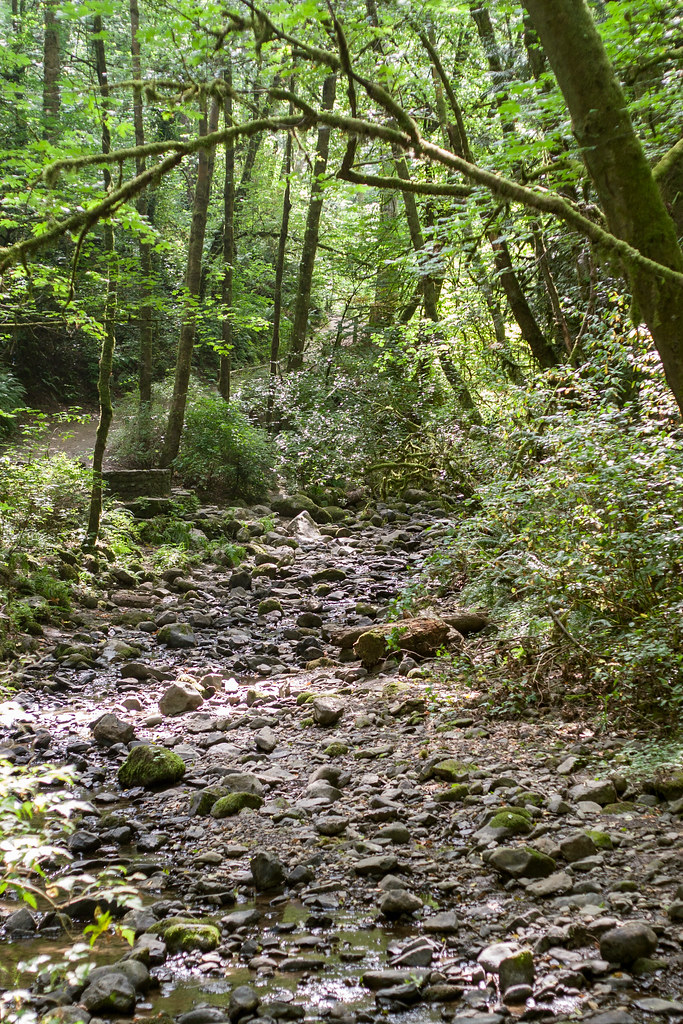
Image 28.7. Forest Park in Portland, Oregon. This greenspace contains a mixture of natural and cultural values for residents. It has 5,000 acres of protected natural area and 80 miles of hiking and walking trails. Source: “Forest Park, Portland, OR” by BenGrantham is licensed under CC BY 2.0.
More typically, however, urban habitats are dominated by non-native or invasive species, which were introduced in various ways. Most non-native or invasive plants were introduced in these ways:
- As seeds contained in soil carried by ships as ballast, which was dumped in a port when the cargo was discharged (this was particularly important before the twentieth century)
- As seeds that contaminated the seedstock of crop plants (this is now less of a problem because weed seeds are “cleaned” from commercial seedstock)
- As plants used in agriculture, forestry, or horticulture
Some non-native or invasive plants have found good habitat in urban areas, where they thrive and out-compete native species. These invasive species are an important ecological problem and can be regarded as a kind of “biological pollution.”
Non-native animals are also common in urban areas. Some, such as the house mouse (Mus musculus) and Norway rat (Rattus norvegicus), were introduced accidentally when they escaped from infested ships and cargoes. Others were deliberately introduced. For example, the rock dove (pigeon; Columba livea), starling (Sturnus vulgaris), and house sparrow (Passer domesticus) were introduced by a nineteenth-century society of gentlemen who were dedicated to bringing all of the birds mentioned in the plays of William Shakespeare to North America.
It is important to distinguish the difference between the terms non-native and invasive species. A non-native species is one that does not originate naturally in a specified area and whose introduction was a result of human activity, either intentional or accidental. Not all non-native species are considered invasive. An invasive species is one that actively imposes a negative impact on the general ecosystem, the economy, the health of humans, other animals, or plants and can be native or non-native to the area. Therefore plants or animals that were not originally found in an area (non-native) but pose no larger threat, are not invasive. Additionally, these terms are relative to a specified location as a species may be invasive in one place, non-native in another, and a native species in its place of origin (NPS, 2020).
Many invasive species now have wild, self-maintaining populations in urban areas. Examples of these invasive plants and animals and the ecological problems that are associated with them include the following:
- The dandelion (Taraxacum officinale) is a perennial, herbaceous plant that was originally native to alpine habitat in Europe, but now occurs in temperate regions throughout the world, probably having been introduced in marine ballast. The dandelion is considered an important weed of lawns and pastures.
- Japanese knotweed (Polygonum cuspidatum) is a perennial, herbaceous plant that grows up to 2-m tall and is native to Japan. It has attractive foliage and was widely introduced for horticultural use. It can be invasive in disturbed areas (Image 28.8).
- The sticky touch-me-not (Impatiens glandulifera) is an annual wildflower native to the Himalayas. It was introduced through horticulture and can be invasive in gardens and wetlands.
- Goutweed (Aegopodium variegatum) is a perennial, herbaceous plant native to Eurasia that was widely introduced through horticulture and is invasive in gardens and other disturbed habitats.
- Purple loosestrife (Lythrum salicaria) is a perennial, herbaceous plant of Eurasia that was introduced through ships’ ballast and horticulture and is a serious invader of wetlands (Image 28.9).
- St. John’s wort (Hypericum perforatum) is a perennial, herbaceous plant of Europe that was introduced probably through marine ballast and is now a serious weed of pastures that causes a photosensitivity disease in cattle.
- Norway maple (Acer platanoides) is a native tree of Europe that was introduced for horticultural use. It invades natural hardwood forest.
- The oriental cockroach (Blatta orientalis) is an insect native to eastern Asia. It was probably introduced accidentally with ship cargo and is a serious pest in homes and other places where food is stored.
- The garden snail (Cepaea hortensis) is a terrestrial mollusk native to Europe. It was probably accidentally introduced with marine ballast and is a garden pest in some regions.
- The house mouse and Norway rat are rodents native to Eurasia that were accidentally introduced from ships and are now serious pests in homes and other places where food is stored.
- The starling, rock dove, and house sparrow are songbirds native to Eurasia that were introduced by European settlers in the Americas who were anxious to have familiar species from their homeland. They displace native birds from nesting sites and are invasive pests.
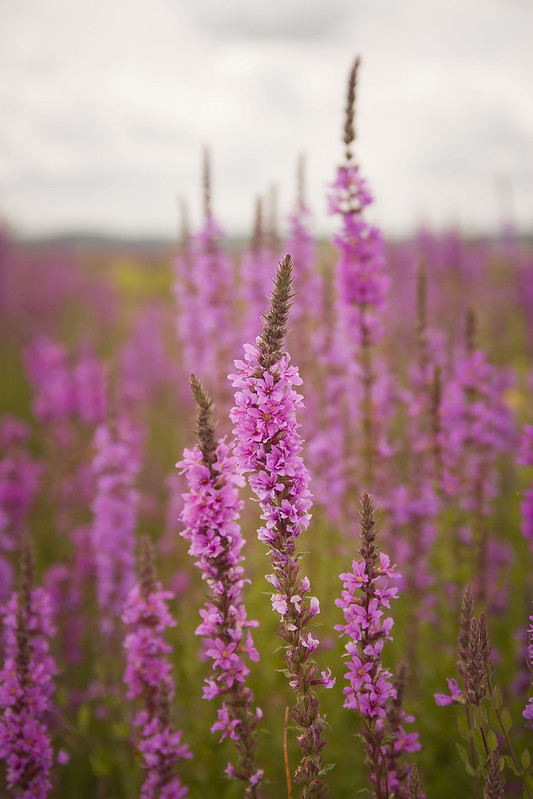
Image 28.8. Purple Loosestrife. Found throughout the entire northern half of the United States, this invasive plant is commonly found in wetland habitats displacing native species. Source: “Purple Loosestrife Blooms” by Sam Stukel, USFWS-Mountain-Prairie, licensed under Public Doman.
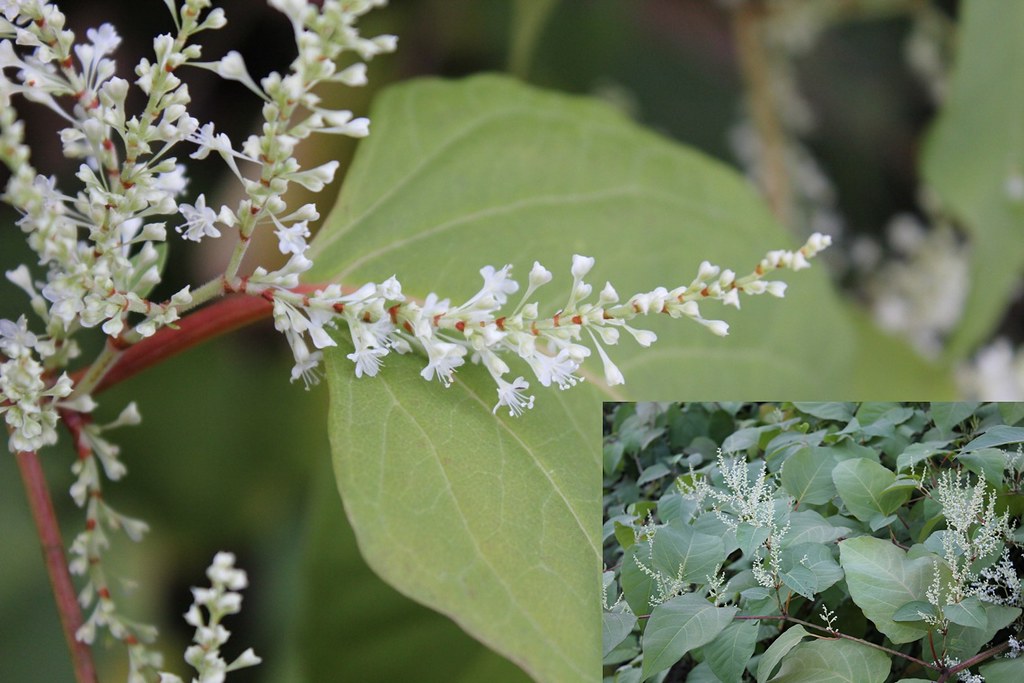
Image 28.9. Japanese Knotweed. Japanese knotweed can spread rapidly once it is introduced to an area and is commonly found along streams, rivers, and roadways. Source: “Japanese Knotweed” by calindarabus is licensed under CC BY 2.0.
Studies on characteristics of urban ecosystems have found that many urban habitats are highly disturbed and are managed to keep them in an early stage of succession. Urban lawns, for example, are mown frequently to prevent their vegetation from developing beyond a stage that is dominated by low-growing, herbaceous plants. Moreover, because most lawn-growers want a monoculture of only one or two species of grasses, they may apply herbicide to kill unwanted dicotyledonous plants, such as clovers, dandelion, and other “weeds.”
The most commonly grown grass in lawns in temperate-zone countries is the Kentucky blue grass (Poa pratensis), which, despite its common name, is actually a Eurasian species. Also cultivated in some regions are ryegrass (Lolium perenne), red fescue (Festuca rubra), and bent grasses (Agrostis species); these are also non-native. The displacement of native plants by non-native ones results in important ecological damage, especially if the foreign ones become invasive. Some ecologists believe that horticulturists should place much greater emphasis on the use of native plants, rather than risk the serious problems associated with biological “pollution” by non-native species. Other aspects of the intensive management of lawns include the use of fertilizer, insecticide, and irrigation. Some ecologists recommend less-intensive systems of lawn management, including tolerance of such non-grass plants as red clover (Trifolium pratense), black medic (Medicago lupulina), and other legumes, which fix atmospheric nitrogen and so reduce the need for fertilizer. Even such species as dandelion and buttercup (Ranunculus acris) can be tolerated in lawns and viewed as attractive “wildflowers” rather than as “weeds.”
The urban forest is another prominent habitat in cities and towns and provides many useful ecological services. For example, large amounts of carbon are stored in urban trees, which helps offset some emissions of CO2 from the combustion of fossil fuels. Urban parks and well-treed residential areas can store as much carbon in tree biomass as can a natural forest. In addition, well-placed trees reduce the wind speed near buildings, which decreases the air-infiltration rate and thereby helps to conserve heat during cold weather. Trees can also shade buildings, reducing the energy needed for air conditioning during warm weather. Transpiration from tree foliage also helps to cool the ambient urban atmosphere, as does the shading of streets, lawns, and other areas. Urban trees also absorb some air pollutants (such as SO2 and particulates), help to reduce noise levels, and greatly improve outdoor aesthetics. A well-developed urban forest also provides habitat for lower-growing plants and for animals in built-up areas.
Tree management plans look at the urban forest in residential, institutional, and parkland areas to assess the species composition, age structure, health and condition of the trees, as well as potential threats. Sidewalk trees and urban forests improve the streetscape aesthetic as well as the pedestrian experience by providing shading. Trees in urban environments are usually selected and planted based on their ornamental value, resilience in harsh “unnatural” conditions, and their suitability for the urban environment. This can result in a lack of biodiversity with a few dominating species, as well as a preference for non-native species. For example, in the city of Binghamton, New York, Norway maple encompassed 22% of the city street tree population. The Tree Management Plan for Binghamton, New York recommends that a tree population should follow the “10-20-30” rule where no more than 10% of a population is of the same species, 20% of the same genus, and 30% of the same family. Therefore, the management plan recommends limiting future planting of Norway maple and to instead plant a greater diversity of trees in the city such as river birch (Betula nigra) and eastern redbud (Cercis Canadensis) (Davey Resource Group, 2018). In addition to Binghamton, New York (Image 28.10), many cities, including Houston, Texas (Image 28.11) have tree management plans and tree planting initiatives to help increase overall biodiversity and ecological health of urban areas.
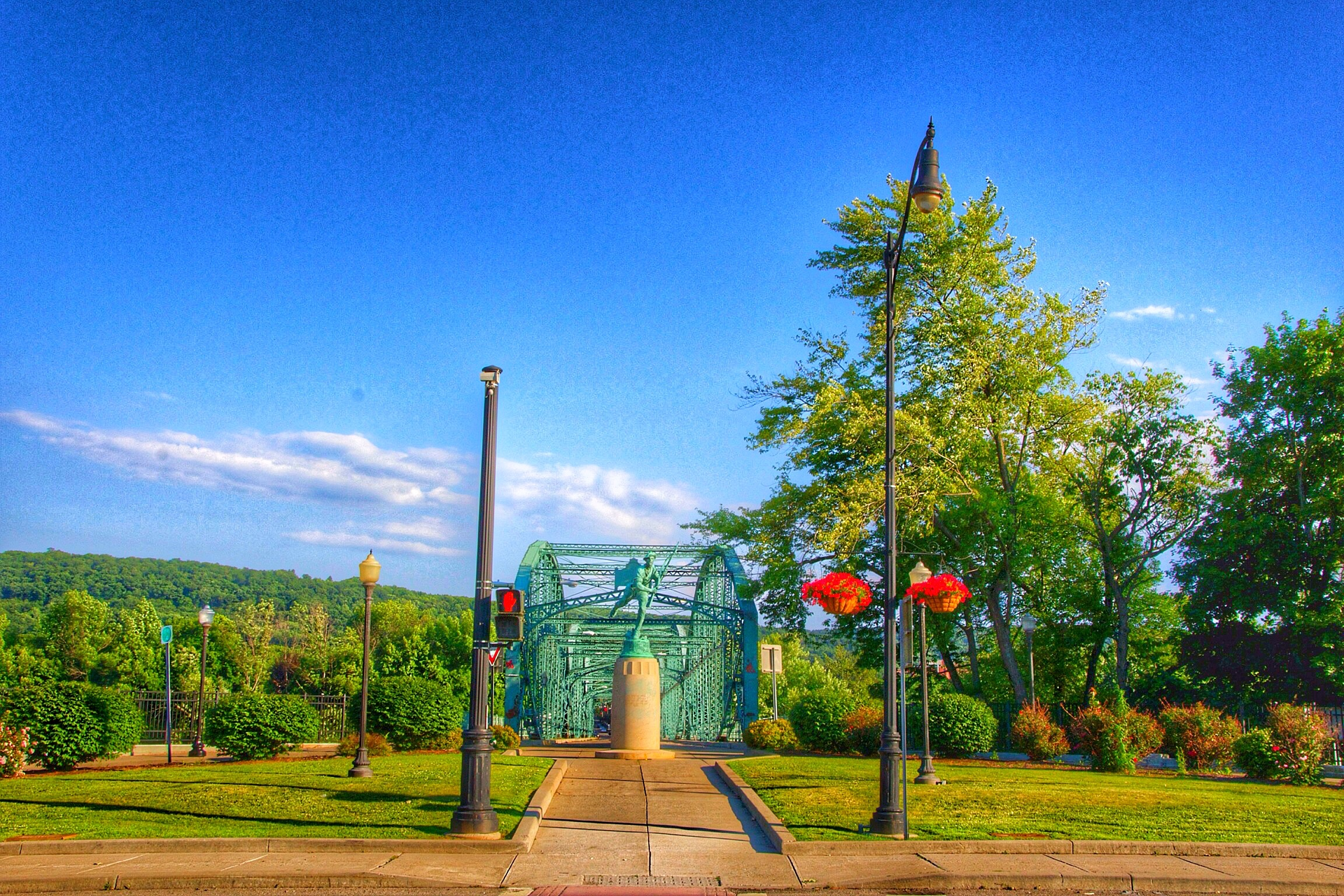
Image 28.10. Urban Forestry in Binghamton, New York. A tree management plan was developed for the City of Binghamton, New York to help in the preservation and continued improvement of the urban forest. “Binghamton New York (Brome County) South Washington Street Bridge” by Onasill ~ Bill is licensed under CC BY-NC-SA 2.0
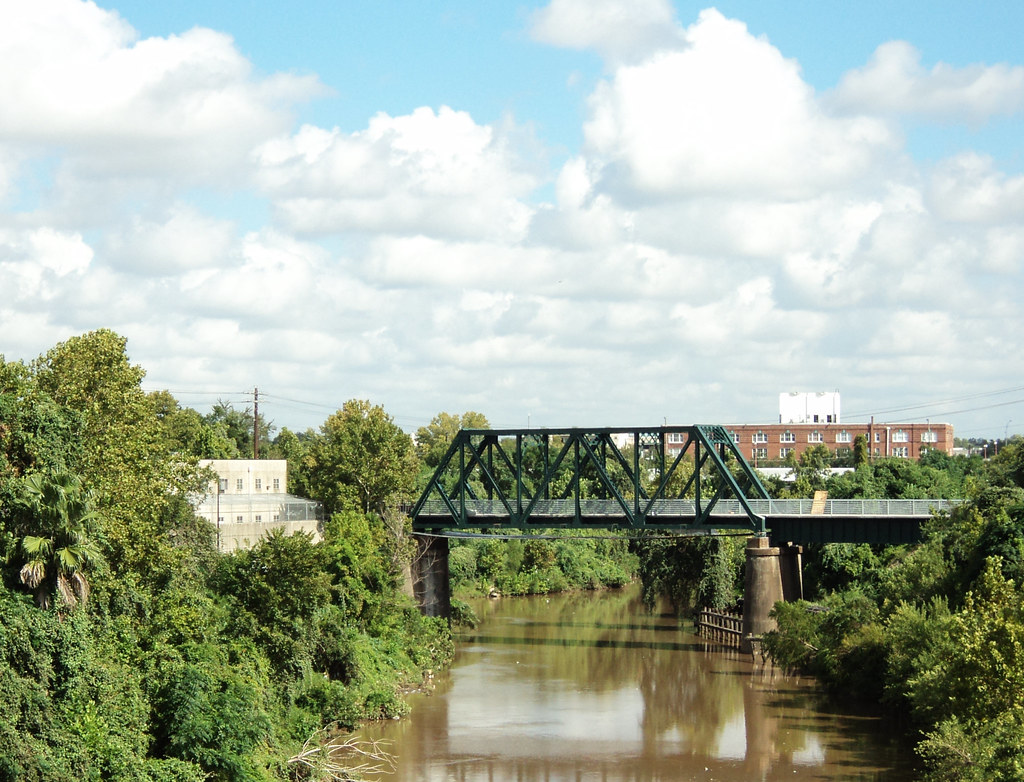
Image 28.11. Forested Area in Houston. Houston has a formidable tree initiative that prioritizes biodiversity and funding for community members who cannot install street trees themselves. “GH&H Railroad Bridge, Buffalo Bayou, Houston, Texas 0911101028” by Patrick Feller is licensed under CC BY 2.0.
Urban environments can also support a variety of bird species, however, usually many of these species are invasive, such as the rock dove, starling, and house sparrow. However, small areas of natural habitat in urban environments can support some native bird populations including American robin (Turdus migratorius), blue jay (Cyanocitta cristata), and cardinal (Cardinalis cardinalis). Waterfowl are also abundant in urban areas with aquatic habitat, such as beside an ocean or near a lake or river. For example, many cities have wild breeding populations of “giant” Canada goose (Branta canadensis maxima), which are descended from birds that were released in those places since the 1950s. The geese find that grassy lawns near water provide suitable feeding habitat, and their population in some cities has increased enormously. However, many of these geese have lost the habit of migrating, and some people consider their abundance and year-round presence to be a nuisance. Some cities have attempted to alleviate their “over-population” of geese by capturing animals and shipping them to willing host cities elsewhere. Some cities are culling the geese by killing part of the population. During the spring and fall migrations, some cities with aquatic habitat support large numbers of native waterfowl and other birds. Some waterfowl may remain during the winter if open water is present.
Some native mammals also occur in urban habitats. Grey (black) squirrels (Sciurus carolinensis) and raccoons (Procyon lotor) may even inhabit downtown neighborhoods as long as there is some open forest available. Urban areas with extensive shrubby and forested habitat may sustain white-tailed deer (Odocoileus virginianus), red fox (Vulpes vulpes), striped skunk (Mephites mephites), and coyote (Canis latrans). The key to sustaining populations of native animals in urban areas is to maintain as much relatively natural habitat as possible, dominated by indigenous plants. If this is done, even inner-city backyards can provide habitat for some native animals. This function is enhanced by the deliberate naturalization of urban habitats by growing native plants in horticulture (see In Detail 28.2).
In Detail 28.2. Urban Naturalization
Urban naturalization is a “new” horticultural practice that favors the use of native plants to achieve pleasing aesthetics in gardening. It is a more natural alternative to conventional horticulture, in which there is a very strong preference for the cultivation of non-native plants. Naturalization avoids many of the ecological problems that are associated with growing non-native species, which can become invasive and damage natural habitats and may be vectors of deadly diseases of native species (see Chapter 14).
But what is meant by terms such as “native” and “natural”? In the sense intended here, and in the context of the Americas, a native (indigenous) species is one that was present in an ecoregion before about 1500 (that is, in pre-Columbian times). If a species was introduced afterward, either deliberately or accidentally, and then developed self-maintaining populations, it would be considered “naturalized” but not indigenous (they may also be “invasive” if they cause ecological damage). “Natural communities” are considered to be self-organizing, co-evolved assemblages of native species that occur in habitats that are appropriate for their survival.
Because many native plants are beautiful and can grow well in habitats provided by gardens, they can be easily used in horticulture. In fact, they may do very well because they are pre-adapted to local climate, soil, and other aspects of the habitat. They may be grown in contrived but pleasing arrangements of flowering plants, shrubs, and trees, as is commonly done in horticulture, or they may be managed to create a facsimile of a natural community.
If naturalization is used to replace conventional horticultural habitats, such as lawns and gardens that are dominated by non-native species, it will result in areas being better suited to support native birds and other animals. This kind of gardening can create beautiful spaces, but it has a much softer ecological footprint. Although naturalized gardens are still uncommon, they are attracting increasing attention from people seeking to express a more natural view of their world.
Conclusions
Urban ecosystems sustain humans, associated non-native organisms, and some native species and remnants of natural habitats. However, urban ecosystems are ecological “islands” that draw upon the surrounding region to continuously supply immense quantities of natural resources and to assimilate wastes. A great challenge for urban ecologists is to develop a better understanding of the structure and function of the urban–industrial techno-ecosystem, including the exchanges of materials and energy and the factors that affect biodiversity. This knowledge can help to identify ecologically pathological relationships, which can then be mitigated to reduce the urban–industrial footprint. If this is done, then modern cities can become more ecologically sustainable than is now the case.
Questions for Review
- What changes were important in the development of urban places during the socio-cultural evolution of our species?
- What is an ecological footprint? What are its key components?
- Characterize the urban ecosystem of your community in terms of its structural (such as species and habitats) and functional (resource use, waste generation) attributes.
- What are major differences between urban and rural biodiversity?
Questions for Discussion
- Make a list of factors that are important in the ecological footprint of your community. How might these be changed in order to decrease the size of the footprint?
- Why do so few people use a bicycle as their routine means of transportation, in spite of its mechanical efficiency and low cost?
- How does your lifestyle contribute to pollution of your environment?
- Define the following terms: dumping (disposal), incineration, recycling, composting, reuse, and waste production and prevention. What role does each of these play in waste management in your community?
- What are the major practices used to treat urban sewage?
- Characterize the principal elements of urban biodiversity in your neighborhood. How could habitats be naturalized in order to support more indigenous species?
- Use the footprint calculator provided by the Global Footprint Network (an environmental organization) to calculate your ecological footprint. (Go to http://www.footprintnetwork.org/en/index.php/GFN/page/personal_footprint/) Try doing a “virtual experiment” by varying aspects of your lifestyle in the calculator to see what effect it has on your personal footprint. For example, see what happens if you ride a bike, use public transit, or fly in an airplane.
Exploring Issues
- Your municipal government has hired you to provide advice as it seeks ways to make the community function in a less damaging, more sustainable manner. What studies would you undertake to determine ways by which urban functions (such as transportation, sewage treatment, and management of solid materials and wastes), land-use patterns, and biodiversity can be improved to contribute to the goal of sustainability? What do you think your recommendations would be?
References Cited and Further Reading
Berkowitz, A.R., C.H. Nilon, and K.S. Hollweg. 2002. Understanding Urban Ecosystems. Springer Verlag, Berlin, Germany.
Breuste, J., H. Feldmann, and O. Ohlmann (eds.). 1998. Urban Ecology. Springer Verlag, Berlin, Germany.
Brinkhoff, T. 2015. Major Agglomerations of the World. http://www.citypopulation.de/world/Agglomerations.html. Accessed June 17, 2021.
Davey Resource Group. 2018. Tree Management Plan: City of Binghamton, New York. https://www.binghamton-ny.gov/home/showdocument?id=1110. Accessed June 17, 2021.
Environmental Protection Agency. 2004. Primer for Municipal Wastewater Treatment Systems. Office of Wastewater Management. Washington D.C. https://www.epa.gov/sites/production/files/2015-09/documents/primer.pdf. Accessed June 17, 2021.
Environmental Protection Agency. 2018. Facts and Figures about Materials, Waste and Recycling: MSW Generated by Material, 2018. https://www.epa.gov/facts-and-figures-about-materials-waste-and-recycling/guide-facts-and-figures-report-about-materials#Products. Accessed June 17, 2021.
Ewing, B., D. Moore, A. Ourser, A. Reed, and M. Wackernagel. 2010. Ecological Footprint Atlas 2010. Global Footprint Network, Oakland, CA. https://www.footprintnetwork.org/content/images/uploads/Ecological_Footprint_Atlas_2010.pdf. Accessed June 17, 2021.
Fincher, R. and J.M. Jacobs (eds.). 1998. Cities of Difference. Guilford Press, New York, NY.
Global Footprint Network. 2017. Ecological Footprint of Countries. Open Data Platform: 2021 National Footprint and Biocapacity Amounts. https://data.footprintnetwork.org/?_ga=2.213014356.193975080.1607636931-1228825067.1607467693#/compareCountries?type=EFCpc&cn=all&yr=2017. Accessed June 17, 2021.
Holcomb, M.C. 1987. Transportation Energy Data Book, 9th ed. Oak Ridge National Laboratory, Oak Ridge, TN.
Jacobs, J. 1961. The Death and Life of Great American Cities. Random House, New York, NY.
Jacobs, J. 1969. The Economy of Cities. Random House, New York, NY.
Kreith, F. (ed.). 1994. Handbook of Solid Waste Management. McGraw-Hill, New York, NY.
Kryter, K.D. 1985. The Effects of Noise. 2nd ed. Academic Press, Orlando, FL.
Landsberg, H.E. 1981. The Urban Climate. Academic Press, New York, NY.
Langford, T.E.L. 1990. Ecological Effects of Thermal Discharges. Elsevier Science, Amsterdam, The Netherlands.
Lilly, C. 2012. Florida Senator Ellyn Bogdanoff and Miami-Dade Mayor Carlos Gimenez Battle Over Everglades Urban Development Boundary. Huffington Post. March 5. https://www.huffpost.com/entry/bogdanoff-udb-amendment_n_1321113. Accessed June 17, 2021.
Metropolitan Transit Authority (MTA). 2020. Subway and Bus Ridership for 2019. New York City Transit. https://new.mta.info/agency/new-york-city-transit/subway-bus-ridership-2019. Accessed June 17, 2021.
National Highway Traffic Safety Administration (NHTSA). 2019. 2018 Fatal Motor Vehicle Crashes: Overview. United States Department of Transportation. https://crashstats.nhtsa.dot.gov/Api/Public/ViewPublication/812826. Accessed June 17, 2021.
National Park Service (NPS). 2020. Invasive & Non-Native Species. https://www.nps.gov/subjects/invasive/learn.htm. Accessed June 17, 2021.
New York City Department of Environmental Protection (NYC DEP). 2018. Summary of the New York City Noise Code. https://www1.nyc.gov/site/dep/environment/noise-code.page. Accessed June 28, 2021.
Platt, R.H., R.A. Rowntree, and P.C. Muick. 1994. The Ecological City: Preserving and Restoring Urban Biodiversity. University of Massachusetts Press Amherst, MA.
Ponting, C. 1991. A Green History of the World. Sinclair-Stevenson London, UK.
Rees, W.E. 2010. The Human Nature of Unsustainability. The Post Carbon Institute, Santa Rosa, CA.
Timerson, B.J. 1999. A Guide to Noise Control in Minnesota. Minnesota Pollution Control Agency. Minneapolis, MN.
Turner, K., L. Lefler, and B. Freedman. 2004. Plant communities of selected urbanized areas of Halifax, Nova Scotia, Canada. Landscape and Urban Planning, 71: 191–206.
USA Facts. 2021. What does America spend on transportation and infrastructure? Is transportation infrastructure improving? https://usafacts.org/state-of-the-union/transportation-infrastructure/. Accessed June 17, 2021.
U.S. Census Bureau. 2010. United States Summary: 2010. 2010 Census of Population and Housing, Population and Housing Unit Counts, CPH-2-5. U.S. Government Printing Office, Washington, DC: 2012. pp. 20–26.
Wackernagel, M. and W.E. Rees. 1996. Our Ecological Footprint: Reducing Human Impact on the Earth. New Society, Gabriola Island, BC.
Vargo, J., B.G. Gerhardstein, G.P. Whitfield, A. Wendel. 2015. Bicyclist deaths associated with motor vehicle traffic – United States, 1975-2012. Morbidity and Mortality Weekly Report, Centers for Disease Control and Prevention, 64(31): 837-841.
World Bank. 2021. DataBank: World Development Indicators. Urban Population (% of total population). https://databank.worldbank.org/reports.aspx?source=2&series=SP.URB.TOTL.IN.ZS#. Accessed June 17, 2021.