Learning Objectives
- Describe the components of the autonomic nervous system
- Differentiate between the structures of the sympathetic and parasympathetic divisions in the autonomic nervous system
- Name the components of a visceral reflex specific to the autonomic division to which it belongs
- Predict the response of a target effector to autonomic input on the basis of the released signaling molecule
- Describe how the central nervous system coordinates and contributes to autonomic functions
- Name the components that generate the sympathetic and parasympathetic responses of the autonomic nervous system
- Explain the differences in output connections within the two divisions of the autonomic nervous system
- Describe the signaling molecules and receptor proteins involved in communication within the two divisions of the autonomic nervous system
- Compare the structure of somatic and autonomic reflex arcs
- Explain the differences in sympathetic and parasympathetic reflexes
- Differentiate between short and long reflexes
- Determine the effect of the autonomic nervous system on the regulation of the various organ systems on the basis of the signaling molecules involved
- Describe the effects of drugs that affect autonomic function
- Describe the role of higher centers of the brain in autonomic regulation
- Explain the connection of the hypothalamus to homeostasis
- Describe the regions of the CNS that link the autonomic system with emotion
- Describe the pathways important to descending control of the autonomic system
- List the classes of pharmaceuticals that interact with the autonomic nervous system
- Differentiate between cholinergic and adrenergic compounds
- Differentiate between sympathomimetic and sympatholytic drugs
- Relate the consequences of nicotine abuse with respect to autonomic control of the cardiovascular system
Introduction to the Autonomic Nervous System
Josie is sitting, having an outdoor lunch with friends when a large spider lands on her plate. She immediately freezes, the food in her mouth begins to feel like a wad of dry hay, and she nearly gags as she tries to swallow it. She feels her heart race and pound in her chest. After swallowing her food, it seems stuck in her throat and chest.
In this scenario, Josie had been sitting, relaxed and enjoying a meal. In this relaxed state, the body would have a heart rate that is at rest, active peristalisis (activity in muscles of the digestive system), ample activity in salivary glands and in digestive gland secretions, and bronchi that are not dilated. In this relaxed state, food can be easily processed due to ample amounts of saliva and digestive enzymes in the saliva released by the salivary glands into the mouth.. Salivation also facilitates swallowing by providing lubrication to the back of the throat and the esophagus. In this relaxed state, digestive fluids and enzymes in the intestines are actively produced and secreted so that food can be further processed and broken down (catabolized) for absorption of nutrients and glucose. While relaxed, Josie’s heart beats imperceptibly and her breathing is deep.
With the sudden appearance of the spider, the rate of Josie’s heart beat becomes more rapid, and it contracts more powerfully. In this vigilant state, Josie senses her rapid heart rate as well as the increased force of the contraction of her heart. She also senses a shift to rapid, shallow breathing that she tries to control. Her food seems lodged near the back of her throat as she struggles to swallow her food safely.
Within seconds of seeing the spider, Josie’s body systems shifted from reflecting calm to a state of panic and hyper-vigilance. These responses to the external threat prepare Josie to either fight or flee the situation. In the hyper-vigilant state, Josie’s pupils widen, she sweats and more blood is pumped through her blood vessels which permits more blood, chemicals, and hormones to flow to her skeletal muscles and respiratory system. As you can see, the effects on organ systems when in either of the states, relaxed or hyper-vigilant, are nearly opposite. These two states are controlled by two subsets of neural pathways that are part of the peripheral nervous system.
The peripheral nervous system itself has two main functional parts. These are the somatic nervous system, which controls voluntary movements of skeletal muscles, and the autonomic nervous system, which is the involuntary motor division of the nervous system.
The autonomic nervous system is further divided into the sympathetic nervous system and the parasympathetic nervous system (Figure 1). It is the complementarity of these two latter branches of the autonomic nervous system that drove the physiological changes in the “Spider Sat Down Beside Her” scenario above.
As evident from the impact of the sight of the spider on Josie’s ability to eat her meal, activity in the parasympathetic system is associated with a relaxing meal; on the other hand, activity in the sympathetic system is associated with alertness and vigilance. In keeping with the complementary functionality of the two systems, they have been given nick names. The parasympathetic branch works for “rest and repose” (also commonly known as “rest and digest”); the sympathetic branch is known for the “fight or flight” response (variously also known as “fight, flight or freeze;” “hyperarousal;” “acute stress”).
It will also be evident in later sections that both parasympathetic and sympathetic branches influence most organs, typically in opposition to each other. However, as will also be clear from the discussion going forward, physiologically this opposition is more complementary than antagonistic. One can give an example of a car, where the accelerator and brake are both necessary for its operation. The balancing that goes on between these two divisions of the ANS requires that most organs receive inputs from both. This is referred to as dual innervation. We will see examples of this later.
Anatomy of Autonomic and Somatic Motor Divisions
In the somatic division of the peripheral nervous system, a single somatic motor neuron whose cell body is in the central nervous system (either in the ventral horn of the spinal cord or in the nucleus of a cranial nerve in the brain) sends an action potential along its axon into the peripheral nervous system until it contacts its target, a skeletal muscle fiber (Figure 2). There, it releases the neurotransmitter acetylcholine (ACh), which binds to nicotinic receptors on the surface of the muscle cell. The result of acetylcholine binding to a nicotinic receptor is always excitatory, so the muscle cell contracts in response to this chemical signal.
The autonomic nervous system has one major structural difference from the somatic, in that there are always two motor neurons (or in one case, two cells) in any signalling pathway used to communicate with an effector organ (Figure 2). The first neuron, called the preganglionic neuron, has its cell body in the central nervous system, either in the brainstem or spinal cord. The preganglionic neuron only carries an action potential along its axon as far as a ganglion in the peripheral nervous system, which contains the cell bodies of postganglionic neurons. At the synapse with the postganglionic neuron, a preganglionic neuron will release ACh, which in turn binds to nicotinic receptors on the cell body and dendrites of the postganglionic neuron. Postganglionic neurons are excited when stimulated with ACh, and generate their own action potential which travels towards the effector organ. At the axon terminal, what happens next depends on which division of the autonomic is active, as well as the receptor on the target organ.
In the case of the parasympathetic division, postganglionic neurons will also secrete ACh at the synapse with the effector (smooth muscle, cardiac muscle, or a gland). The response of the target depends on the receptor it possesses, since muscarinic receptors can be either excited or inhibited by ACh. A salivary gland contacted by ACh would become excited causing one to salivate, but a pacemaker cell in the SA node of the heart would be inhibited by ACh, slowing one’s heart rate.
For the sympathetic division, there is a bit more variety in the cells involved and the neurotransmitters used, specifically there are three different scenarios. Postganglionic neurons that stimulate sweat glands (not pictured in Figure 2) will secrete ACh onto the cells of the sweat glands, which possess muscarinic receptors that are excited by the binding of ACh. In the case of every other postganglionic neuron in the sympathetic division, norepinephrine (NE) is secreted at the synapse with a target cell. Finally, it is possible that the preganglionic neuron doesn’t synapse with a postganglionic neuron at all, and instead synapses in the medulla of the adrenal gland with a chromaffin cell. This endocrine cell secretes E (mostly) and some NE directly into the bloodstream as hormones. As with the parasympathetic division, what happens next depends on the receptor on the target cell.
Adrenergic receptors come in two varieties α and β. α (alpha) receptors have two subtypes: α1 and α2. β (beta) receptors have three subtypes: β1, β2, and β3. NE and E can bind to either of these receptors, but some adrenergic receptors have a higher affinity for one than the other, and the response of the target cell varies depending on the type of receptor that was stimulated. α1 produce excitatory effects when stimulated by either E or NE. α2 act to regulate NE release. β1 receptors have a high affinity for both NE and E, and are found in locations such as the heart and brain. β2 receptors have a low affinity for NE, with a higher affinity for E, and act to promote relaxation of smooth muscles in addition to stimulating many of the metabolic effects associated with E. β3 receptors are only found on adipose tissue, where binding of the receptor stimulates lipolysis.
Dual Innervation
As you might expect, certain organs and tissues receive innervation from both the sympathetic and parasympathetic nervous systems. These organs are said to be “dually innervated.” An example of this is how the parasympathetic division facilitates micturition and defecation, while sympathetic input activates sphincters of the bladder and the rectum, impending micturition and defecation, respectively.
The heart and lungs are dually innervated (Figure 3). Alterations of the pace and the force of contraction of heart muscles are influenced by the effect of the vagus nerve on the pacemaker cells of the heart. Parasympathetic input to both right and left vagus (CN X) nerves provide cervical cardiac nerves to the cardiac plexus. The preganglionic sympathetic fibers to the heart originate from the T1-T4/ T5 of the spinal cord. Normal heart rhythm is in the 60-100 beats per minute range. During restful conditions, the vagus nerve (CN X), through parasympathetic input, has the dominant influence that maintains the heart rate in the 60-80 beats per min (bpm) range. Under stress, excitement, or exertion, sympathetic stimulation can shift the heart rate to greater than 100 bpm. A slower than normal heart rate, less than 60 bpm, mediated by vagus nerve and the parasympathetic nervous system or an illness, is referred to as bradycardia. A faster than normal heart rate can be mediated by sympathetic nervous systems or can be due to illness, and is referred to as tachycardia.
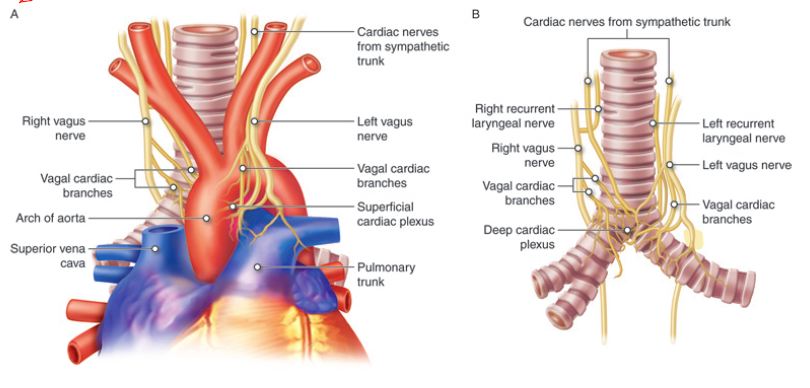
Figure 3. Dual innervation of the heart.
For lung innervation, the sympathetic and parasympathetic divisions of the autonomic nervous system nerves are arranged as the pulmonary plexi and innervate the smooth muscle and glands of the bronchi and pulmonary blood vessels. The sympathetic preganglionic fibers to the lungs arise from T1-T4. The sympathetic stimulation of the bronchial smooth muscles is achieved by the epinephrine released from adrenal glands. Overall, the sympathetic stimulation causes vasoconstriction and bronchodilation in the lungs. Stimulation by parasympathetic input causes vasodilation and bronchoconstriction in the lungs.
Everybody is familiar with asthma. Whatever the causality, asthma is characterized by bronchoconstriction due to increased sensitivity to irritating stimuli (which could be due to allergic response to certain antigens or due to irritants in the environment like coal dust, exhaust fumes, etc.). Increased mucus secretions rapid bronchoconstriction leads to labored breathing which we know as “asthma.”
Sympathetic and Parasympathetic Effectors
There are certain effectors in your body that are not dually innervated. Sweat glands, arrector pili muscles, adrenal medula, liver, adipocytes, lacrymal glands, radial muscle of the iris, juxtaglomerular apparatus, uterus and most vascular smooth muscles have only sympathetic innervation. In contrast to the sympathetic system, there are relatively few organs that function only with parasympathetic stimulation. Examples of such organs are the circular muscle of iris which causes pupillary constriction and the parietal cells of the stomach that secrete gastric acid.
Most vasculature of smooth muscles receive only sympathetic innervations. The balance of vascular constriction and dilation is mediated by sympathetic tone. The tone of a single vessel is proportional to the sympathetic stimulation it is receiving. More stimulation leads to more constriction, and, as sympathetic input to the vessel decreases, the vessel relaxes. Also, it is important to recognize that the impact of the release of epinephrine or norepinephrine on blood vessels is dependent on the subtype of neurotransmitter receptor on those blood vessels. For example, sympathetic activation leads to constriction of most vasculature in the body (mediated through stimulation of alpha-type receptors), but leads to dilation of coronary vessels supplying the heart (mediated through stimulation of beta-type receptors). The difference in how the vessel responds is due to the type of adrenergic receptor, and, ultimately, what type of intracellular signaling pathways are engaged to drive the vascular response. Adrenergic receptors are stimulated by either norepinephrine or epinephrine.
Most vessels in the body have the alpha-type receptor for binding norepinephrine, which results in vasoconstriction. Vasculature of the heart, on the other hand, has beta-type receptors for binding norepinephrine, which results in vasodilation. Also, recall that sympathetic nervous system activation causes release of epinephrine by chromaffin cells of adrenal medullae. Epinephrine has a greater effect on stimulating beta receptors than does norepinephrine, which means that epinephrine has a stronger effect on cardiac stimulation and a much weaker effect on blood vessels in muscles. Stimulation of beta receptors in the heart causes dilation of coronary blood vessels supplying the heart, increase heart rate, and increase in the strength of contraction of cardiac muscle. Blood vessels in skeletal muscles express alpha and beta adrenergic receptors. During exercise, blood vessels in skeletal muscle dilate, and, among many possible factors (ATP, lactic acid, carbon dioxide, oxygen, adenosine) that could cause this vasodilation, circulating epinephrine released into the bloodstream by chromaffin cells in the adrenal medullae is one other possible factor.
Chemical Signaling in the Autonomic Nervous System
Where an autonomic neuron connects with a target, there is a synapse. The electrical signal of the action potential causes the release of a signaling molecule, which will bind to receptor proteins on the target cell. Synapses of the autonomic system are classified as either cholinergic, meaning that acetylcholine (ACh) is released, or adrenergic, meaning that norepinephrine is released. The terms cholinergic and adrenergic refer not only to the signaling molecule that is released but also to the class of receptors that each binds.
The cholinergic system includes two classes of receptor: the nicotinic receptor and the muscarinic receptor. Both receptor types bind to ACh and cause changes in the target cell. The nicotinic receptor is a ligand-gated cation channel and the muscarinic receptor is a G protein–coupled receptor. The receptors are named for, and differentiated by, other molecules that bind to them. Whereas nicotine will bind to the nicotinic receptor, and muscarine will bind to the muscarinic receptor, there is no cross-reactivity between the receptors. The situation is similar to locks and keys.
Imagine two locks—one for a classroom and the other for an office—that are opened by two separate keys. The classroom key will not open the office door and the office key will not open the classroom door. This is similar to the specificity of nicotine and muscarine for their receptors. However, a master key can open multiple locks, such as a master key for the Biology Department that opens both the classroom and the office doors. This is similar to ACh that binds to both types of receptors. The molecules that define these receptors are not crucial—they are simply tools for researchers to use in the laboratory. These molecules are exogenous, meaning that they are made outside of the human body, so a researcher can use them without any confounding endogenous results (results caused by the molecules produced in the body).
The adrenergic system also has two types of receptors, named the alpha (α)-adrenergic receptor and beta (β)-adrenergic receptor. Unlike cholinergic receptors, these receptor types are not classified by which drugs can bind to them. All of them are G protein–coupled receptors. There are three types of α-adrenergic receptors, termed α1, α2, and α3, and there are two types of β-adrenergic receptors, termed β1 and β2. An itional aspect of the adrenergic system is that there is a second signaling molecule called epinephrine. The chemical difference between norepinephrine and epinephrine is the addition of a methyl group (CH3) in epinephrine. The prefix “nor-” actually refers to this chemical difference, in which a methyl group is missing.
The term adrenergic should remind you of the word adrenaline, which is associated with the fight-or-flight response described at the beginning of the chapter. Adrenaline and epinephrine are two names for the same molecule. The adrenal gland (in Latin, ad– = “on top of”; renal = “kidney”) secretes adrenaline. The ending “-ine” refers to the chemical being derived, or extracted, from the adrenal gland. A similar construction from Greek instead of Latin results in the word epinephrine (epi– = “above”; nephr– = “kidney”). In scientific usage, epinephrine is preferred in the United States, whereas adrenaline is preferred in Great Britain, because “adrenalin” was once a registered, proprietary drug name in the United States. Though the drug is no longer sold, the convention of referring to this molecule by the two different names persists. Similarly, norepinephrine and noradrenaline are two names for the same molecule.
Having understood the cholinergic and adrenergic systems, their role in the autonomic system is relatively simple to understand. All preganglionic fibers, both sympathetic and parasympathetic, release ACh. All ganglionic neurons—the targets of these preganglionic fibers—have nicotinic receptors in their cell membranes. The nicotinic receptor is a ligand-gated cation channel that results in depolarization of the postsynaptic membrane. The postganglionic parasympathetic fibers also release ACh, but the receptors on their targets are muscarinic receptors, which are G protein–coupled receptors and do not exclusively cause depolarization of the postsynaptic membrane. Postganglionic sympathetic fibers release norepinephrine, except for fibers that project to sweat glands and to blood vessels associated with skeletal muscles, which release ACh (Table 1).
Table 1 | ||
---|---|---|
Sympathetic | Parasympathetic | |
Preganglionic | Acetylcholine > nicotinic receptor | Acetylcholine > nicotinic receptor |
Postganglionic | Norepinephrine > a or B-adrenergic receptors Acetylcholine > muscarinic receptor (associated with sweat glands and the blood vessels associated with skeletal muscles only |
Acetylcholine > muscarinic receptor |
Signaling molecules can belong to two broad groups. Neurotransmitters are released at synapses, whereas hormones are released into the bloodstream. These are simplistic definitions, but they can help to clarify this point. Acetylcholine can be considered a neurotransmitter because it is released by axons at synapses. The adrenergic system, however, presents a challenge. Postganglionic sympathetic fibers release norepinephrine, which can be considered a neurotransmitter. But the adrenal medulla releases epinephrine and norepinephrine into circulation, so they should be considered hormones.
What are referred to here as synapses may not fit the strictest definition of synapse. Some sources will refer to the connection between a postganglionic fiber and a target effector as neuroeffector junctions; neurotransmitters, as defined above, would be called neuromodulators. The structure of postganglionic connections are not the typical synaptic end bulb that is found at the neuromuscular junction, but rather are chains of swellings along the length of a postganglionic fiber called a varicosity (Figure 4).
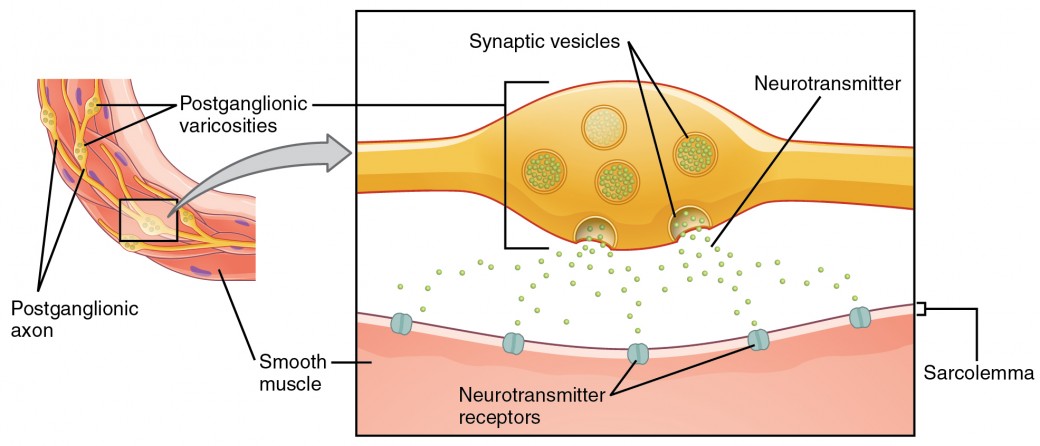
Figure 4. Autonomic Varicosities. The connection between autonomic fibers and target effectors is not the same as the typical synapse, such as the neuromuscular junction. Instead of a synaptic end bulb, a neurotransmitter is released from swellings along the length of a fiber that makes an extended network of connections in the target effector.
Candela Citations
- Anatomy & Physiology. Authored by: OpenStax College. Provided by: Rice University. Located at: http://cnx.org/contents/14fb4ad7-39a1-4eee-ab6e-3ef2482e3e22@9.1. License: CC BY: Attribution. License Terms: Download for free at http://cnx.org/contents/14fb4ad7-39a1-4eee-ab6e-3ef2482e3e22@9.1
- Unit 14: Nervous System (Module 55). Authored by: Open Learning Initiative. Provided by: Carnegie Mellon University. Located at: https://oli.cmu.edu/jcourse/workbook/activity/page?context=4348992480020ca600aaf40f3acfbc21. Project: Anatomy & Physiology. License: CC BY-NC-SA: Attribution-NonCommercial-ShareAlike