Introduction to Synapses
Several neurons are generally needed to transmit a signal from one place in the body to another. So how does the signal pass from one neuron to the next along a neural pathway? The signal must be transmitted across the interface between successive neurons and we will learn how that is accomplished in this next section.
The term synapse means “coming together.” Where two structures or entities come together, they form a synapse. Although one can use the word synapse to mean any cellular junction, in physiology we traditionally limit its usage to: the junction of two neurons, the junction between a neuron and a target cell (ex. the neuromuscular junction), or the interface between adjacent cardiac muscle cells or adjacent smooth muscle cells. In the nervous system, a synapse is the structure that allows a neuron to pass an electrical or chemical signal to another cell.
Synapse Cells
The cell that delivers the signal to the synapse is the presynaptic cell. The cell that will receive the signal once it crosses the synapse is the postsynaptic cell. Since most neural pathways contain several neurons, a postsynaptic neuron at one synapse may become the presynaptic neuron for another cell downstream.
A presynaptic neuron can form one of three types of synapses with a postsynaptic neuron. The most common type of synapse is an axodendritic synapse, where the axon of the presynaptic neuron synapses with a dendrite of the postsynaptic neuron. If the presynpatic neuron synapses with the soma of the postsynaptic neuron it is called an axosomatic synapse, and if it synapses with the axon of the postsynaptic cell it is an axoaxonic synapse. Although our illustration shows a single synapse, neurons typically have many (even 10,000 or more) synapses.
Synapses Types
There are two types of synapses found in your body: electrical and chemical (Figure 1). Electrical synapses allow the direct passage of ions and signaling molecules from cell to cell. In contrast, chemical synapses do not pass the signal directly from the presynaptic cell to the postsynaptic cell. In a chemical synapse, an action potential in the presynaptic neuron leads to the release of a chemical messenger called a neurotransmitter. The neurotransmitter then diffuses across the synapse and binds to receptors on the postsynaptic cell. Binding of the neurotransmitter leads to the production of an electrical signal in the postsynaptic cell.
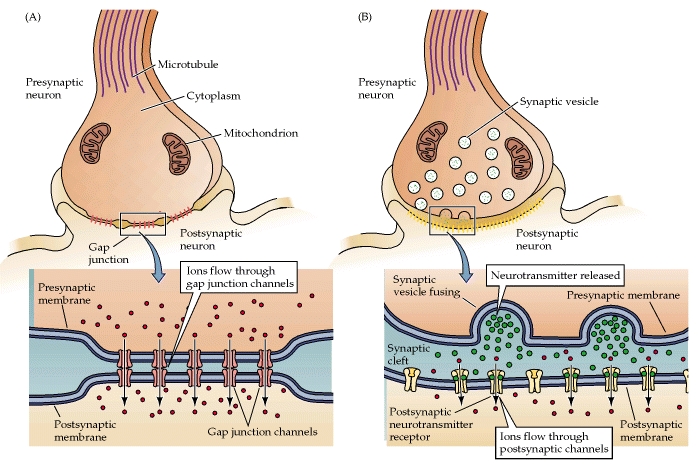
Figure 1. A) Electrical synapses allow ions to diffuse through gap junctions from the pre to the postsynaptic cell. B) At a chemical synapse, a presynaptic neuron releases neurotransmitter towards the postsynaptic cell.
Why does the body have two types of synapses? Each type of synapse has functional advantages and disadvantages. An electrical synapse passes the signal very quickly, which allows groups of cells to act in unison. A chemical synapse takes much longer to transmit the signal from one cell to the next; however, chemical synapses allow neurons to integrate information from multiple presynaptic neurons, determining whether or not the postsynaptic cell will continue to propagate the signal. Neurons respond differently based on information transmitted by multiple chemical synapses. Let’s take a closer look at the structure and function of each type of synapse.
Electrical synapses transmit action potentials via the direct flow of electrical current at gap junctions (Figure 2). Gap junctions are formed when two adjacent cells have transmembrane pores that align. The membranes of the two cells are linked together and the aligned pores form a passage between the cells. Consequently, several types of molecules and ions are allowed to pass between the cells. Due to the direct flow of ions and molecules from one cell to another, electrical synapses allow bidirectional flow of information between cells. Gap junctions are crucial to the functioning of the cardiac myocytes and smooth muscles.
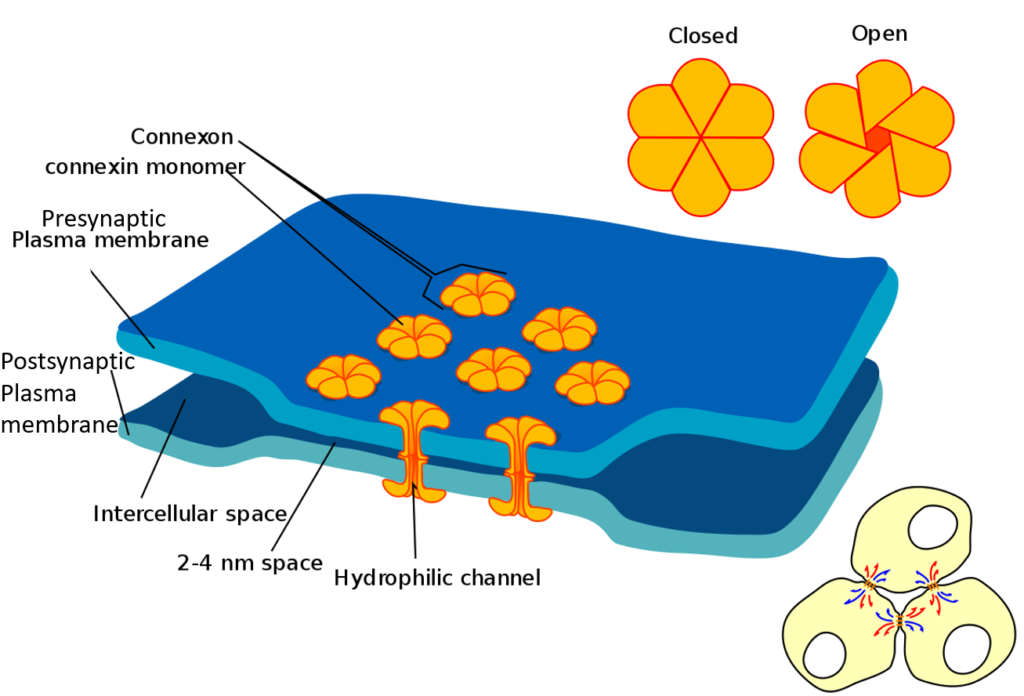
Figure 2. The intracellular fluid of the pre and postsynaptic cells are linked by protein channels called connexons.
Chemical synapses comprise most of the synapses in your body (Figure 3). In a chemical synapse, a synaptic gap or cleft separates the pre- and the postsynaptic cells. An action potential propagated to the axon terminal results in the secretion of chemical messengers, called neurotransmitters, from the axon terminals. The neurotransmitter molecules diffuse across the synaptic cleft and bind to receptor proteins on the cell membrane of the postsynaptic cell. Binding of the neurotransmitter to the receptors on the postsynaptic cell leads to a transient change in the postsynaptic cell’s membrane potential.
An example of a chemical synapse is the neuromuscular junction (NMJ) described in the chapter on muscle tissue. In the nervous system, there are many more synapses that are essentially the same as the NMJ. All synapses have common characteristics, which can be summarized in this list:
- presynaptic element
- neurotransmitter (packaged in vesicles)
- synaptic cleft
- receptor proteins
- postsynaptic element
- neurotransmitter elimination or re-uptake
For the NMJ, these characteristics are as follows: the presynaptic element is the motor neuron’s axon terminals, the neurotransmitter is acetylcholine, the synaptic cleft is the space between the cells where the neurotransmitter diffuses, the receptor protein is the nicotinic acetylcholine receptor, the postsynaptic element is the sarcolemma of the muscle cell, and the neurotransmitter is eliminated by acetylcholinesterase. Other synapses are similar to this, and the specifics are different, but they all contain the same characteristics.
Neurotransmitter Release
When an action potential reaches the axon terminals, voltage-gated Ca2+ channels in the membrane of the synaptic end bulb open. The concentration of Ca2+ increases inside the end bulb, and the Ca2+ ion associates with proteins in the outer surface of neurotransmitter vesicles. The Ca2+ facilitates the merging of the vesicle with the presynaptic membrane so that the neurotransmitter is released through exocytosis into the small gap between the cells, known as the synaptic cleft.
Once in the synaptic cleft, the neurotransmitter diffuses the short distance to the postsynaptic membrane and can interact with neurotransmitter receptors. Receptors are specific for the neurotransmitter, and the two fit together like a key and lock. One neurotransmitter binds to its receptor and will not bind to receptors for other neurotransmitters, making the binding a specific chemical event (Figure 4).
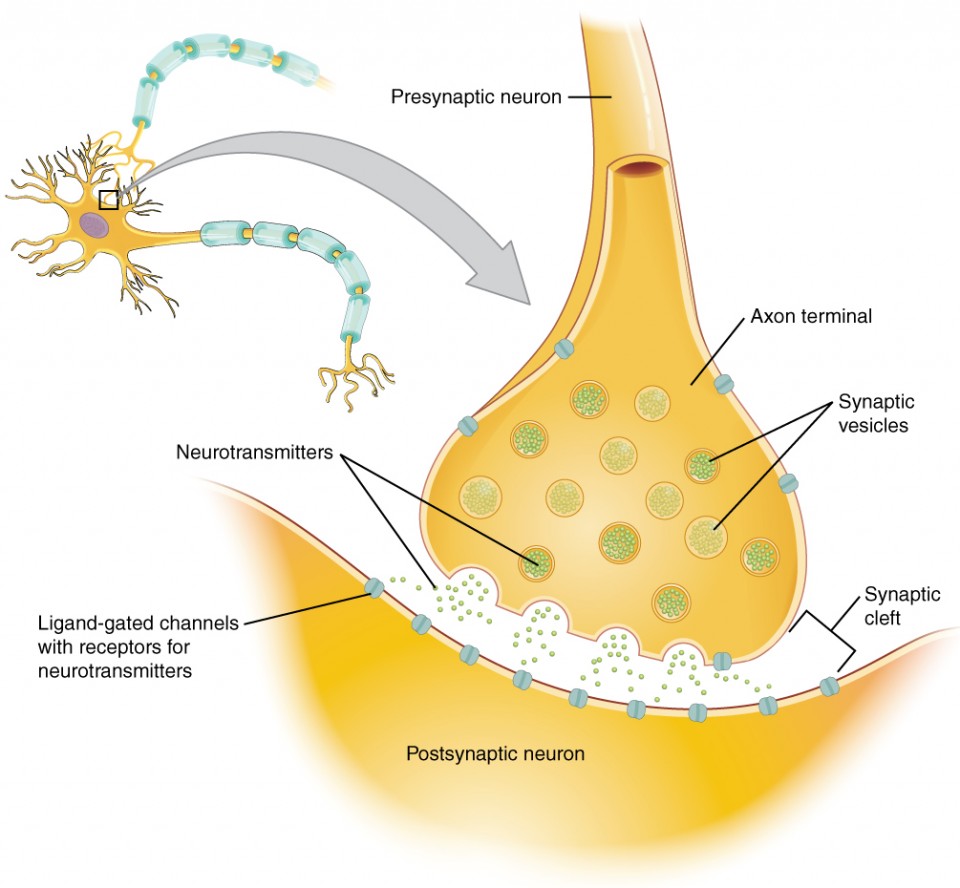
Figure 4. The Synapse. The synapse is a connection between a neuron and its target cell (which is not necessarily a neuron). The presynaptic element is the synaptic end bulb of the axon where Ca2+ enters the bulb to cause vesicle fusion and neurotransmitter release. The neurotransmitter diffuses across the synaptic cleft to bind to its receptor. The neurotransmitter is cleared from the synapse either by enzymatic degradation, neuronal reuptake, or glial reuptake.
Watch this video to learn about the release of a neurotransmitter.
The action potential reaches the end of the axon, called the axon terminal, and a chemical signal is released to tell the target cell to do something—either to initiate a new action potential, or to suppress that activity. In a very short space, the electrical signal of the action potential is changed into the chemical signal of a neurotransmitter and then back to electrical changes in the target cell membrane. What is the importance of voltage-gated calcium channels in the release of neurotransmitters?
Disorders of the Nervous System
The underlying cause of some neurodegenerative diseases, such as Alzheimer’s and Parkinson’s, appears to be related to proteins—specifically, to proteins behaving badly. One of the strongest theories of what causes Alzheimer’s disease is based on the accumulation of beta-amyloid plaques, dense conglomerations of a protein that is not functioning correctly. Parkinson’s disease is linked to an increase in a protein known as alpha-synuclein that is toxic to the cells of the substantia nigra nucleus in the midbrain.
For proteins to function correctly, they are dependent on their three-dimensional shape. The linear sequence of amino acids folds into a three-dimensional shape that is based on the interactions between and among those amino acids. When the folding is disturbed, and proteins take on a different shape, they stop functioning correctly. But the disease is not necessarily the result of functional loss of these proteins; rather, these altered proteins start to accumulate and may become toxic. For example, in Alzheimer’s, the hallmark of the disease is the accumulation of these amyloid plaques in the cerebral cortex. The term coined to describe this sort of disease is “proteopathy” and it includes other diseases. Creutzfeld-Jacob disease, the human variant of the prion disease known as mad cow disease in the bovine, also involves the accumulation of amyloid plaques, similar to Alzheimer’s. Diseases of other organ systems can fall into this group as well, such as cystic fibrosis or type 2 diabetes. Recognizing the relationship between these diseases has suggested new therapeutic possibilities. Interfering with the accumulation of the proteins, and possibly as early as their original production within the cell, may unlock new ways to alleviate these devastating diseases.
Self-Check Questions
Take the quiz below to check your understanding of Communication Between Neurons: