Learning Objectives
- Describe how enzymes in body fluids provide protection against infection or disease
- List and describe the function of antimicrobial peptides, complement components, cytokines, and acute-phase proteins
- Describe similarities and differences among classic, alternate, and lectin complement pathways
In addition to physical defenses, the innate nonspecific immune system uses a number of chemical mediators that inhibit microbial invaders. The term “chemical mediators” encompasses a wide array of substances found in various body fluids and tissues throughout the body. Chemical mediators may work alone or in conjunction with each other to inhibit microbial colonization and infection.
Some chemical mediators are endogenously produced, meaning they are produced by human body cells; others are produced exogenously, meaning that they are produced by certain microbes that are part of the microbiome. Some mediators are produced continually, bathing the area in the antimicrobial substance; others are produced or activated primarily in response to some stimulus, such as the presence of microbes.
Chemical and Enzymatic Mediators Found in Body Fluids
Fluids produced by the skin include examples of both endogenous and exogenous mediators. Sebaceous glands in the dermis secrete an oil called sebum that is released onto the skin surface through hair follicles. This sebum is an endogenous mediator, providing an additional layer of defense by helping seal off the pore of the hair follicle, preventing bacteria on the skin’s surface from invading sweat glands and surrounding tissue (Figure 1). Certain members of the microbiome, such as the bacterium Propionibacterium acnes and the fungus Malassezia, among others, can use lipase enzymes to degrade sebum, using it as a food source. This produces oleic acid, which creates a mildly acidic environment on the surface of the skin that is inhospitable to many pathogenic microbes. Oleic acid is an example of an exogenously produced mediator because it is produced by resident microbes and not directly by body cells.
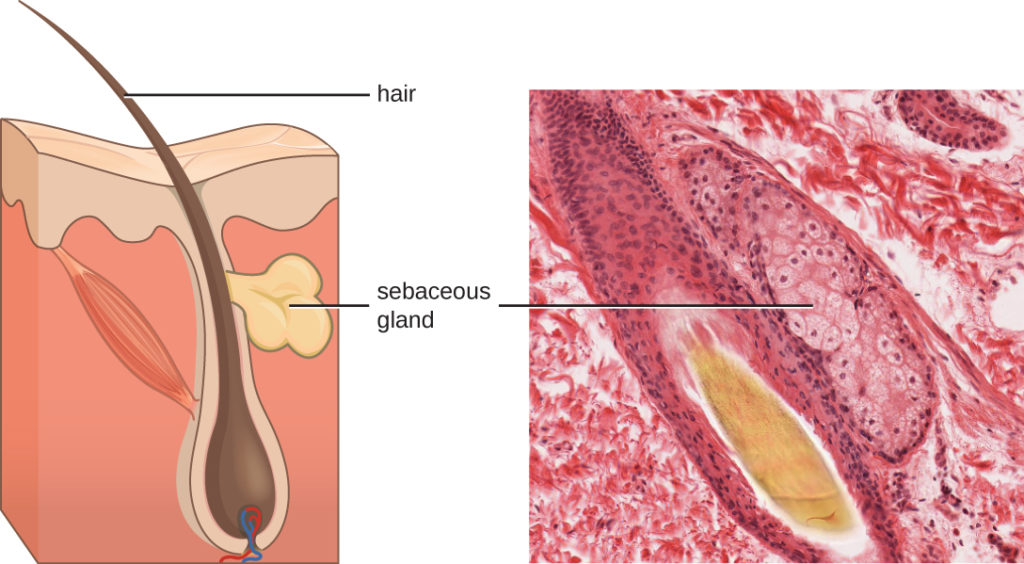
Figure 1. Sebaceous glands secrete sebum, a chemical mediator that lubricates and protect the skin from invading microbes. Sebum is also a food source for resident microbes that produce oleic acid, an exogenously produced mediator. (credit micrograph: Micrograph provided by the Regents of University of Michigan Medical School © 2012)
Environmental factors that affect the microbiota of the skin can have a direct impact on the production of chemical mediators. Low humidity or decreased sebum production, for example, could make the skin less habitable for microbes that produce oleic acid, thus making the skin more susceptible to pathogens normally inhibited by the skin’s low pH. Many skin moisturizers are formulated to counter such effects by restoring moisture and essential oils to the skin.
The digestive tract also produces a large number of chemical mediators that inhibit or kill microbes. In the oral cavity, saliva contains mediators such as lactoperoxidase enzymes, and mucus secreted by the esophagus contains the antibacterial enzyme lysozyme. In the stomach, highly acidic gastric fluid kills most microbes. In the lower digestive tract, the intestines have pancreatic and intestinal enzymes, antibacterial peptides (cryptins), bile produced from the liver, and specialized Paneth cells that produce lysozyme. Together, these mediators are able to eliminate most pathogens that manage to survive the acidic environment of the stomach.
In the urinary tract, urine flushes microbes out of the body during urination. Furthermore, the slight acidity of urine (the average pH is about 6) inhibits the growth of many microbes and potential pathogens in the urinary tract.
The female reproductive system employs lactate, an exogenously produced chemical mediator, to inhibit microbial growth. The cells and tissue layers composing the vagina produce glycogen, a branched and more complex polymer of glucose. Lactobacilli in the area ferment glycogen to produce lactate, lowering the pH in the vagina and inhibiting transient microbiota, opportunistic pathogens like Candida (a yeast associated with vaginal infections), and other pathogens responsible for sexually transmitted diseases.
In the eyes, tears contain the chemical mediators lysozyme and lactoferrin, both of which are capable of eliminating microbes that have found their way to the surface of the eyes. Lysozyme cleaves the bond between NAG and NAM in peptidoglycan, a component of the cell wall in bacteria. It is more effective against gram-positive bacteria, which lack the protective outer membrane associated with gram-negative bacteria. Lactoferrin inhibits microbial growth by chemically binding and sequestering iron. This effectually starves many microbes that require iron for growth.
In the ears, cerumen (earwax) exhibits antimicrobial properties due to the presence of fatty acids, which lower the pH to between 3 and 5.
The respiratory tract uses various chemical mediators in the nasal passages, trachea, and lungs. The mucus produced in the nasal passages contains a mix of antimicrobial molecules similar to those found in tears and saliva (e.g., lysozyme, lactoferrin, lactoperoxidase). Secretions in the trachea and lungs also contain lysozyme and lactoferrin, as well as a diverse group of additional chemical mediators, such as the lipoprotein complex called surfactant, which has antibacterial properties.
Think about It
- Explain the difference between endogenous and exogenous mediators
- Describe how pH affects antimicrobial defenses
Antimicrobial Peptides
The antimicrobial peptides (AMPs) are a special class of nonspecific cell-derived mediators with broad-spectrum antimicrobial properties. Some AMPs are produced routinely by the body, whereas others are primarily produced (or produced in greater quantities) in response to the presence of an invading pathogen. Research has begun exploring how AMPs can be used in the diagnosis and treatment of disease.
AMPs may induce cell damage in microorganisms in a variety of ways, including by inflicting damage to membranes, destroying DNA and RNA, or interfering with cell-wall synthesis. Depending on the specific antimicrobial mechanism, a particular AMP may inhibit only certain groups of microbes (e.g., gram-positive or gram-negative bacteria) or it may be more broadly effective against bacteria, fungi, protozoa, and viruses. Many AMPs are found on the skin, but they can also be found in other regions of the body.
A family of AMPs called defensins can be produced by epithelial cells throughout the body as well as by cellular defenses such as macrophages and neutrophils (see Cellular Defenses). Defensins may be secreted or act inside host cells; they combat microorganisms by damaging their plasma membranes. AMPs called bacteriocins are produced exogenously by certain members of the resident microbiota within the gastrointestinal tract. The genes coding for these types of AMPs are often carried on plasmids and can be passed between different species within the resident microbiota through lateral or horizontal gene transfer.
There are numerous other AMPs throughout the body. The characteristics of a few of the more significant AMPs are summarized in Table 1.
Table 1. Characteristics of Selected Antimicrobial Peptides (AMPs) | ||||
---|---|---|---|---|
AMP | Secreted by | Body site | Pathogens inhibited | Mode of action |
Bacteriocins | Resident microbiota | Gastrointestinal tract | Bacteria | Disrupt membrane |
Cathelicidin | Epithelial cells, macrophages, and other cell types | Skin | Bacteria and fungi | Disrupts membrane |
Defensins | Epithelial cells, macrophages, neutrophils | Throughout the body | Fungi, bacteria, and many viruses | Disrupt membrane |
Dermicidin | Sweat glands | Skin | Bacteria and fungi | Disrupts membrane integrity and ion channels |
Histatins | Salivary glands | Oral cavity | Fungi | Disrupt intracellular function |
Plasma Protein Mediators
Many nonspecific innate immune factors are found in plasma, the fluid portion of blood. Plasma contains electrolytes, sugars, lipids, and proteins, each of which helps to maintain homeostasis (i.e., stable internal body functioning), and contains the proteins involved in the clotting of blood. Additional proteins found in blood plasma, such as acute-phase proteins, complement proteins, and cytokines, are involved in the nonspecific innate immune response.
Plasma versus Serum
There are two terms for the fluid portion of blood: plasma and serum. How do they differ if they are both fluid and lack cells? The fluid portion of blood left over after coagulation (blood cell clotting) has taken place is serum. Although molecules such as many vitamins, electrolytes, certain sugars, complement proteins, and antibodies are still present in serum, clotting factors are largely depleted. Plasma, conversely, still contains all the clotting elements. To obtain plasma from blood, an anticoagulant must be used to prevent clotting. Examples of anticoagulants include heparin and ethylene diamine tetraacetic acid (EDTA). Because clotting is inhibited, once obtained, the sample must be gently spun down in a centrifuge. The heavier, denser blood cells form a pellet at the bottom of a centrifuge tube, while the fluid plasma portion, which is lighter and less dense, remains above the cell pellet.
Acute-Phase Proteins
The acute-phase proteins are another class of antimicrobial mediators. Acute-phase proteins are primarily produced in the liver and secreted into the blood in response to inflammatory molecules from the immune system. Examples of acute-phase proteins include C-reactive protein, serum amyloid A, ferritin, transferrin, fibrinogen, and mannose-binding lectin. Each of these proteins has a different chemical structure and inhibits or destroys microbes in some way (Table 2).
Table 2. Some Acute-Phase Proteins and Their Functions | |
---|---|
C-reactive protein | Coats bacteria (opsonization), preparing them for ingestion by phagocytes |
Serum amyloid A | |
Ferritin | Bind and sequester iron, thereby inhibiting the growth of pathogens |
Transferrin | |
Fibrinogen | Involved in formation of blood clots that trap bacterial pathogens |
Mannose-binding lectin | Activates complement cascade |
The Complement System
The complement system is a group of plasma protein mediators that can act as an innate nonspecific defense while also serving to connect innate and adaptive immunity (discussed in the next chapter). The complement system is composed of more than 30 proteins (including C1 through C9) that normally circulate as precursor proteins in blood. These precursor proteins become activated when stimulated or triggered by a variety of factors, including the presence of microorganisms. Complement proteins are considered part of innate nonspecific immunity because they are always present in the blood and tissue fluids, allowing them to be activated quickly. Also, when activated through the alternative pathway (described later in this section), complement proteins target pathogens in a nonspecific manner.
The process by which circulating complement precursors become functional is called complement activation. This process is a cascade that can be triggered by one of three different mechanisms, known as the alternative, classical, and lectin pathways.
The alternative pathway is initiated by the spontaneous activation of the complement protein C3. The hydrolysis of C3 produces two products, C3a and C3b. When no invader microbes are present, C3b is very quickly degraded in a hydrolysis reaction using the water in the blood. However, if invading microbes are present, C3b attaches to the surface of these microbes. Once attached, C3b will recruit other complement proteins in a cascade (Figure 2).
The classical pathway provides a more efficient mechanism of activating the complement cascade, but it depends upon the production of antibodies by the specific adaptive immune defenses. To initiate the classical pathway, a specific antibody must first bind to the pathogen to form an antibody-antigen complex. This activates the first protein in the complement cascade, the C1 complex. The C1 complex is a multipart protein complex, and each component participates in the full activation of the overall complex. Following recruitment and activation of the C1 complex, the remaining classical pathway complement proteins are recruited and activated in a cascading sequence (Figure 2).
The lectin activation pathway is similar to the classical pathway, but it is triggered by the binding of mannose-binding lectin, an acute-phase protein, to carbohydrates on the microbial surface. Like other acute-phase proteins, lectins are produced by liver cells and are commonly upregulated in response to inflammatory signals received by the body during an infection (Figure 2).
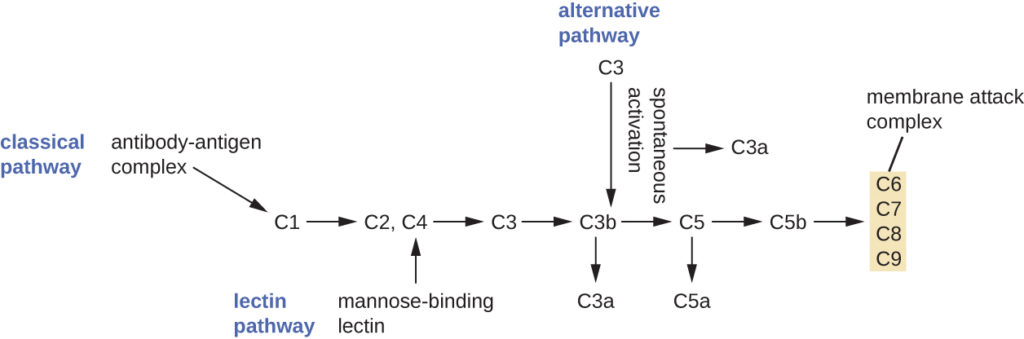
Figure 2. The three complement activation pathways have different triggers, as shown here, but all three result in the activation of the complement protein C3, which produces C3a and C3b. The latter binds to the surface of the target cell and then works with other complement proteins to cleave C5 into C5a and C5b. C5b also binds to the cell surface and then recruits C6 through C9; these molecules form a ring structure called the membrane attack complex (MAC), which punches through the cell membrane of the invading pathogen, causing it to swell and burst.
Although each complement activation pathway is initiated in a different way, they all provide the same protective outcomes: opsonization, inflammation, chemotaxis, and cytolysis. The term opsonization refers to the coating of a pathogen by a chemical substance (called an opsonin) that allows phagocytic cells to recognize, engulf, and destroy it more easily. Opsonins from the complement cascade include C1q, C3b, and C4b. Additional important opsonins include mannose-binding proteins and antibodies. The complement fragments C3a and C5a are well-characterized anaphylatoxins with potent proinflammatory functions. Anaphylatoxins activate mast cells, causing degranulation and the release of inflammatory chemical signals, including mediators that cause vasodilation and increased vascular permeability. C5a is also one of the most potent chemoattractants for neutrophils and other white blood cells, cellular defenses that will be discussed in the next section.
The complement proteins C6, C7, C8, and C9 assemble into a membrane attack complex (MAC), which allows C9 to polymerize into pores in the membranes of gram-negative bacteria. These pores allow water, ions, and other molecules to move freely in and out of the targeted cells, eventually leading to cell lysis and death of the pathogen (Figure 2). However, the MAC is only effective against gram-negative bacteria; it cannot penetrate the thick layer of peptidoglycan associated with cell walls of gram-positive bacteria. Since the MAC does not pose a lethal threat to gram-positive bacterial pathogens, complement-mediated opsonization is more important for their clearance.
Cytokines
Cytokines are soluble proteins that act as communication signals between cells. In a nonspecific innate immune response, various cytokines may be released to stimulate production of chemical mediators or other cell functions, such as cell proliferation, cell differentiation, inhibition of cell division, apoptosis, and chemotaxis.
When a cytokine binds to its target receptor, the effect can vary widely depending on the type of cytokine and the type of cell or receptor to which it has bound. The function of a particular cytokine can be described as autocrine, paracrine, or endocrine (Figure 3). In autocrine function, the same cell that releases the cytokine is the recipient of the signal; in other words, autocrine function is a form of self-stimulation by a cell. In contrast, paracrine function involves the release of cytokines from one cell to other nearby cells, stimulating some response from the recipient cells. Last, endocrine function occurs when cells release cytokines into the bloodstream to be carried to target cells much farther away.
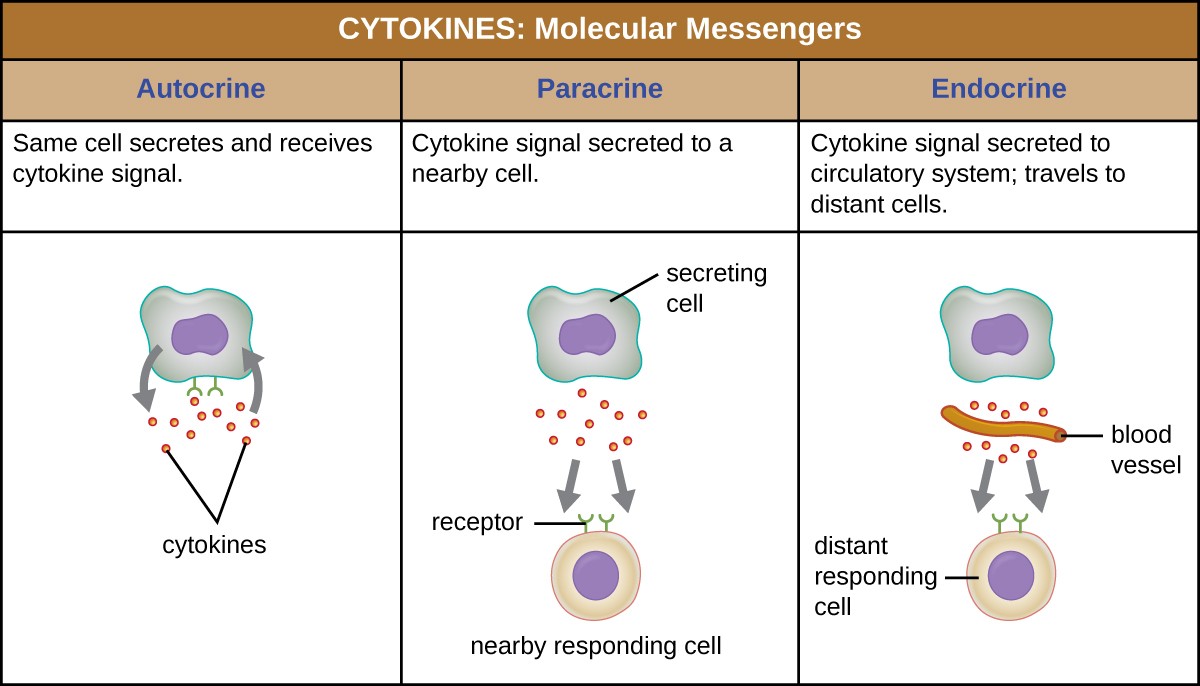
Figure 3. Autocrine, paracrine, and endocrine actions describe which cells are targeted by cytokines and how far the cytokines must travel to bind to their intended target cells’ receptors.
Three important classes of cytokines are the interleukins, chemokines, and interferons. The interleukins were originally thought to be produced only by leukocytes (white blood cells) and to only stimulate leukocytes, thus the reasons for their name. Although interleukins are involved in modulating almost every function of the immune system, their role in the body is not restricted to immunity. Interleukins are also produced by and stimulate a variety of cells unrelated to immune defenses.
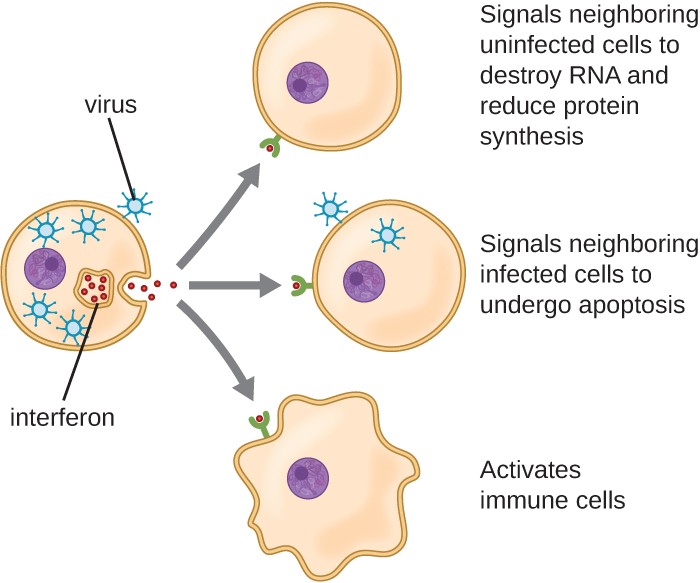
Figure 4. Click for a larger image. Interferons are cytokines released by a cell infected with a virus. Interferon-α and interferon-β signal uninfected neighboring cells to inhibit mRNA synthesis, destroy RNA, and reduce protein synthesis (top arrow). Interferon-α and interferon-β also promote apoptosis in cells infected with the virus (middle arrow). Interferon-γ alerts neighboring immune cells to an attack (bottom arrow). Although interferons do not cure the cell releasing them or other infected cells, which will soon die, their release may prevent additional cells from becoming infected, thus stemming the infection.
The chemokines are chemotactic factors that recruit leukocytes to sites of infection, tissue damage, and inflammation. In contrast to more general chemotactic factors, like complement factor C5a, chemokines are very specific in the subsets of leukocytes they recruit.
Interferons are a diverse group of immune signaling molecules and are especially important in our defense against viruses. Type I interferons (interferon-α and interferon-β) are produced and released by cells infected with virus. These interferons stimulate nearby cells to stop production of mRNA, destroy RNA already produced, and reduce protein synthesis. These cellular changes inhibit viral replication and production of mature virus, slowing the spread of the virus. Type I interferons also stimulate various immune cells involved in viral clearance to more aggressively attack virus-infected cells. Type II interferon (interferon-γ) is an important activator of immune cells (Figure 4).
Inflammation-Eliciting Mediators
Many of the chemical mediators discussed in this section contribute in some way to inflammation and fever, which are nonspecific immune responses discussed in more detail in Inflammation and Fever. Cytokines stimulate the production of acute-phase proteins such as C-reactive protein and mannose-binding lectin in the liver. These acute-phase proteins act as opsonins, activating complement cascades through the lectin pathway.
Some cytokines also bind mast cells and basophils, inducing them to release histamine, a proinflammatory compound. Histamine receptors are found on a variety of cells and mediate proinflammatory events, such as bronchoconstriction (tightening of the airways) and smooth muscle contraction.
In addition to histamine, mast cells may release other chemical mediators, such as leukotrienes. Leukotrienes are lipid-based proinflammatory mediators that are produced from the metabolism of arachidonic acid in the cell membrane of leukocytes and tissue cells. Compared with the proinflammatory effects of histamine, those of leukotrienes are more potent and longer lasting. Together, these chemical mediators can induce coughing, vomiting, and diarrhea, which serve to expel pathogens from the body.
Certain cytokines also stimulate the production of prostaglandins, chemical mediators that promote the inflammatory effects of kinins and histamines. Prostaglandins can also help to set the body temperature higher, leading to fever, which promotes the activities of white blood cells and slightly inhibits the growth of pathogenic microbes (see Inflammation and Fever).
Another inflammatory mediator, bradykinin, contributes to edema, which occurs when fluids and leukocytes leak out of the bloodstream and into tissues. It binds to receptors on cells in the capillary walls, causing the capillaries to dilate and become more permeable to fluids.
Think about It
- What do the three complement activation pathways have in common?
- Explain autocrine, paracrine, and endocrine signals.
- Name two important inflammation-eliciting mediators.
Table 3 provides a summary of the chemical defenses discussed in this section.
Table 3. Chemical Defenses of Nonspecific Innate Immunity | ||
---|---|---|
Defense | Examples | Function |
Chemicals and enzymes in body fluids | Sebum from sebaceous glands | Provides oil barrier protecting hair follicle pores from pathogens |
Oleic acid from sebum and skin microbiota | Lowers pH to inhibit pathogens | |
Lysozyme in secretions | Kills bacteria by attacking cell wall | |
Acid in stomach, urine, and vagina | Inhibits or kills bacteria | |
Digestive enzymes and bile | Kill bacteria | |
Lactoferrin and transferrin | Bind and sequester iron, inhibiting bacterial growth | |
Surfactant in lungs | Kills bacteria | |
Antimicrobial peptides | Defensins, bacteriocins, dermicidin, cathelicidin, histatins, | Kill bacteria by attacking membranes or interfering with cell functions |
Plasma protein mediators | Acute-phase proteins (C-reactive protein, serum amyloid A, ferritin, fibrinogen, transferrin, and mannose-binding lectin) | Inhibit the growth of bacteria and assist in the trapping and killing of bacteria |
Complements C3b and C4b | Opsonization of pathogens to aid phagocytosis | |
Complement C5a | Chemoattractant for phagocytes | |
Complements C3a and C5a | Proinflammatory anaphylatoxins | |
Cytokines | Interleukins | Stimulate and modulate most functions of immune system |
Chemokines | Recruit white blood cells to infected area | |
Interferons | Alert cells to viral infection, induce apoptosis of virus-infected cells, induce antiviral defenses in infected and nearby uninfected cells, stimulate immune cells to attack virus-infected cells | |
Inflammation-eliciting mediators | Histamine | Promotes vasodilation, bronchoconstriction, smooth muscle contraction, increased secretion and mucus production |
Leukotrienes | Promote inflammation; stronger and longer lasting than histamine | |
Prostaglandins | Promote inflammation and fever | |
Bradykinin | Increases vasodilation and vascular permeability, leading to edema |
Key Concepts and Summary
- Numerous chemical mediators produced endogenously and exogenously exhibit nonspecific antimicrobial functions.
- Many chemical mediators are found in body fluids such as sebum, saliva, mucus, gastric and intestinal fluids, urine, tears, cerumen, and vaginal secretions.
- Antimicrobial peptides (AMPs) found on the skin and in other areas of the body are largely produced in response to the presence of pathogens. These include dermcidin, cathelicidin, defensins, histatins, and bacteriocins.
- Plasma contains various proteins that serve as chemical mediators, including acute-phase proteins, complement proteins, and cytokines.
- The complement system involves numerous precursor proteins that circulate in plasma. These proteins become activated in a cascading sequence in the presence of microbes, resulting in the opsonization of pathogens, chemoattraction of leukocytes, induction of inflammation, and cytolysis through the formation of a membrane attack complex (MAC).
- Cytokines are proteins that facilitate various nonspecific responses by innate immune cells, including production of other chemical mediators, cell proliferation, cell death, and differentiation.
- Cytokines play a key role in the inflammatory response, triggering production of inflammation-eliciting mediators such as acute-phase proteins, histamine, leukotrienes, prostaglandins, and bradykinin.
Multiple Choice
Which of the following serve as chemical signals between cells and stimulate a wide range of nonspecific defenses?
- cytokines
- antimicrobial peptides
- complement proteins
- antibodies
Bacteriocins and defensins are types of which of the following?
- leukotrienes
- cytokines
- inflammation-eliciting mediators
- antimicrobial peptides
Which of the following chemical mediators is secreted onto the surface of the skin?
- cerumen
- sebum
- gastric acid
- prostaglandin
Identify the complement activation pathway that is triggered by the binding of an acute-phase protein to a pathogen.
- classical
- alternate
- lectin
- cathelicidin
Histamine, leukotrienes, prostaglandins, and bradykinin are examples of which of the following?
- chemical mediators primarily found in the digestive system
- chemical mediators that promote inflammation
- antimicrobial peptides found on the skin
- complement proteins that form MACs
Fill in the Blank
________ are antimicrobial peptides produced by members of the normal microbiota.
________ is the fluid portion of a blood sample that has been drawn in the presence of an anticoagulant compound.
The process by which cells are drawn or attracted to an area by a microbe invader is known as ________.
Think about It
Differentiate the main activation methods of the classic, alternative, and lectin complement cascades.
What are the four protective outcomes of complement activation?