Learning Objectives
- Explain the distinguishing characteristics of eukaryotic cells
- Describe internal and external structures of prokaryotic cells in terms of their physical structure, chemical structure, and function
- Identify and describe structures and organelles unique to eukaryotic cells
- Compare and contrast similar structures found in prokaryotic and eukaryotic cells
Eukaryotic organisms include protozoans, algae, fungi, plants, and animals. Some eukaryotic cells are independent, single-celled microorganisms, whereas others are part of multicellular organisms. The cells of eukaryotic organisms have several distinguishing characteristics. Above all, eukaryotic cells are defined by the presence of a nucleus surrounded by a complex nuclear membrane. Also, eukaryotic cells are characterized by the presence of membrane-bound organelles in the cytoplasm. Organelles such as mitochondria, the endoplasmic reticulum (ER), Golgi apparatus, lysosomes, and peroxisomes are held in place by the cytoskeleton, an internal network that supports transport of intracellular components and helps maintain cell shape (Figure 1). The genome of eukaryotic cells is packaged in multiple, rod-shaped chromosomes as opposed to the single, circular-shaped chromosome that characterizes most prokaryotic cells. Table 1 compares the characteristics of eukaryotic cell structures with those of bacteria and archaea.
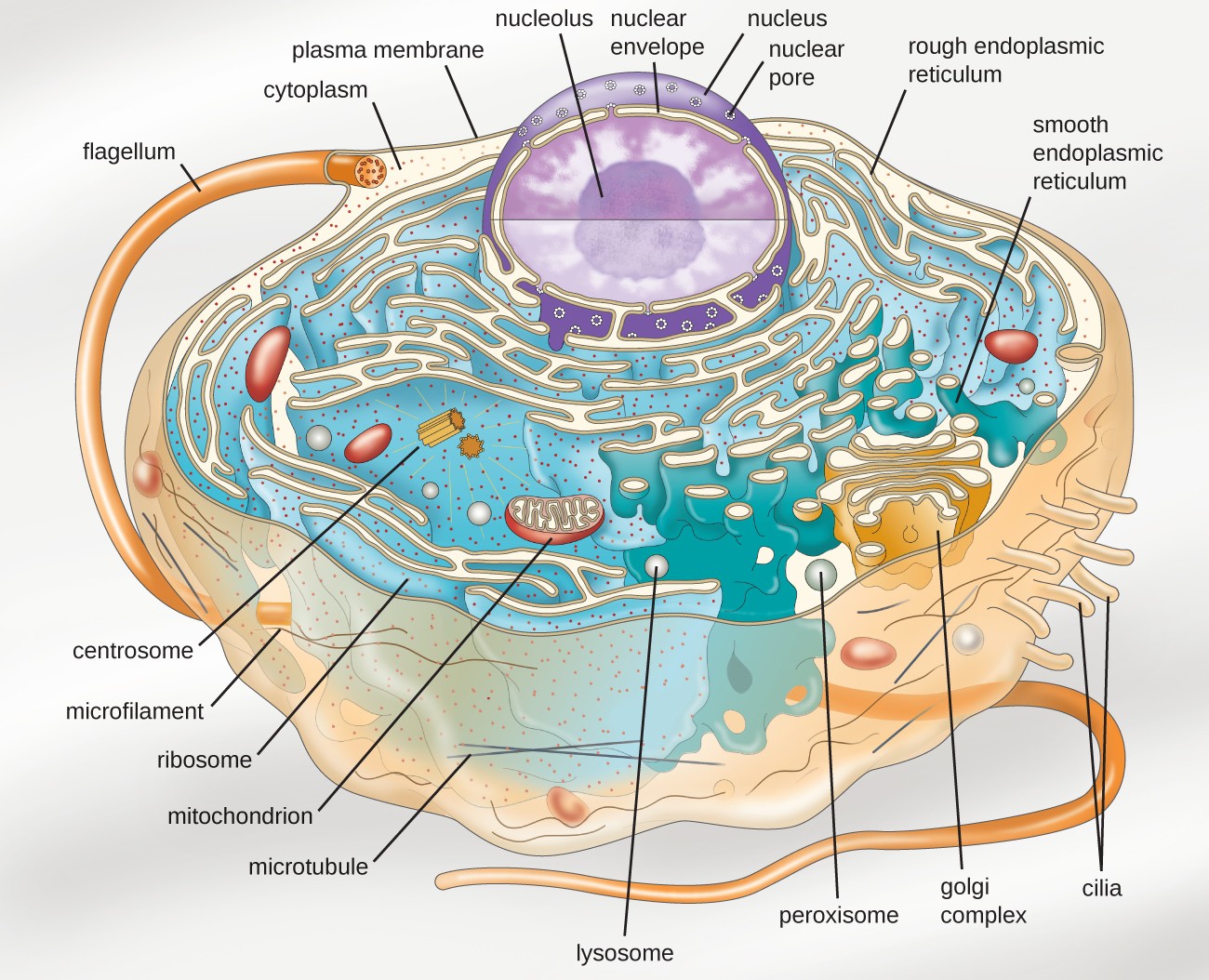
Figure 1. Click for a larger image. An illustration of a generalized, single-celled eukaryotic organism. Note that cells of eukaryotic organisms vary greatly in terms of structure and function, and a particular cell may not have all of the structures shown here.
Table 1. Summary of Cell Structures | |||
---|---|---|---|
Cell Structure | Prokaryotes | Eukaryotes | |
Bacteria | Archaea | ||
Size | ~0.5–1 μM | ~0.5–1 μM | ~5–20 μM |
Surface area-to-volume ratio | High | High | Low |
Nucleus | No | No | Yes |
Genome characteristics |
|
|
|
Cell division | Binary fission | Binary fission | Mitosis, meiosis |
Membrane lipid composition |
|
|
|
Cell wall composition |
|
|
|
Motility structures | Rigid spiral flagella composed of flagellin | Rigid spiral flagella composed of archaeal flagellins | Flexible flagella and cilia composed of microtubules |
Membrane-bound organelles | No | No | Yes |
Endomembrane system | No | No | Yes (ER, Golgi, lysosomes) |
Ribosomes | 70S | 70S |
|
Cell Morphologies
Eukaryotic cells display a wide variety of different cell morphologies. Possible shapes include spheroid, ovoid, cuboidal, cylindrical, flat, lenticular, fusiform, discoidal, crescent, ring stellate, and polygonal (Figure 2). Some eukaryotic cells are irregular in shape, and some are capable of changing shape. The shape of a particular type of eukaryotic cell may be influenced by factors such as its primary function, the organization of its cytoskeleton, the viscosity of its cytoplasm, the rigidity of its cell membrane or cell wall (if it has one), and the physical pressure exerted on it by the surrounding environment and/or adjoining cells.
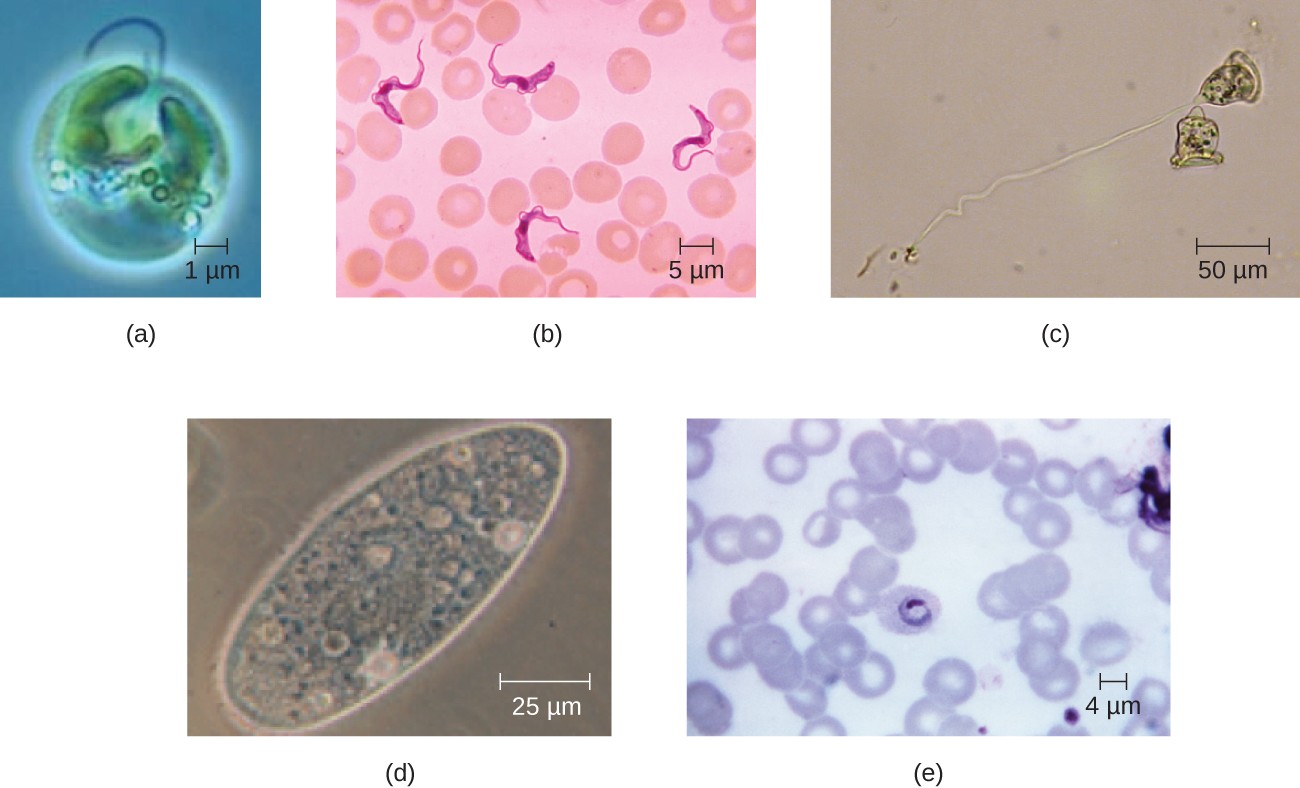
Figure 2. Eukaryotic cells come in a variety of cell shapes. (a) Spheroid Chromulina alga. (b) Fusiform shaped Trypanosoma. (c) Bell-shaped Vorticella. (d) Ovoid Paramecium. (e) Ring-shaped Plasmodium ovale. (credit a: modification of work by NOAA; credit b, e: modification of work by Centers for Disease Control and Prevention)
Think about It
- Identify two differences between eukaryotic and prokaryotic cells.
Nucleus
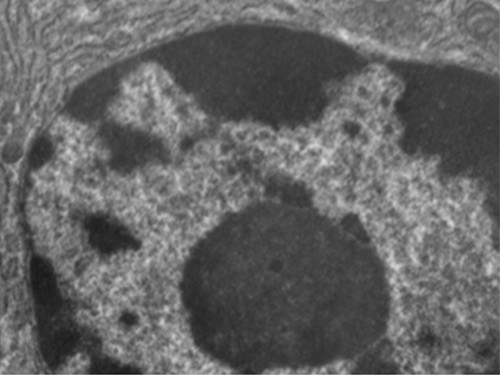
Figure 3. Eukaryotic cells have a well-defined nucleus. The nucleus of this mammalian lung cell is the large, dark, oval-shaped structure in the lower half of the image.
Unlike prokaryotic cells, in which DNA is loosely contained in the nucleoid region, eukaryotic cells possess a nucleus, which is surrounded by a complex nuclear membrane that houses the DNA genome (Figure 3). By containing the cell’s DNA, the nucleus ultimately controls all activities of the cell and also serves an essential role in reproduction and heredity. Eukaryotic cells typically have their DNA organized into multiple linear chromosomes. The DNA within the nucleus is highly organized and condensed to fit inside the nucleus, which is accomplished by wrapping the DNA around proteins called histones.
Although most eukaryotic cells have only one nucleus, exceptions exist. For example, protozoans of the genus Paramecium typically have two complete nuclei: a small nucleus that is used for reproduction (micronucleus) and a large nucleus that directs cellular metabolism (macronucleus). Additionally, some fungi transiently form cells with two nuclei, called heterokaryotic cells, during sexual reproduction. Cells whose nuclei divide, but whose cytoplasm does not, are called coenocytes.
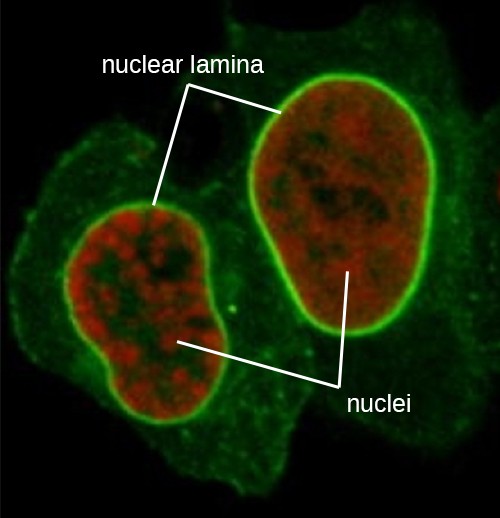
Figure 4. In this fluorescent microscope image, all the intermediate filaments have been stained with a bright green fluorescent stain. The nuclear lamina is the intense bright green ring around the faint red nuclei.
The nucleus is bound by a complex nuclear membrane, often called the nuclear envelope, that consists of two distinct lipid bilayers that are contiguous with each other (Figure 4). Despite these connections between the inner and outer membranes, each membrane contains unique lipids and proteins on its inner and outer surfaces. The nuclear envelope contains nuclear pores, which are large, rosette-shaped protein complexes that control the movement of materials into and out of the nucleus. The overall shape of the nucleus is determined by the nuclear lamina, a meshwork of intermediate filaments found just inside the nuclear envelope membranes. Outside the nucleus, additional intermediate filaments form a looser mesh and serve to anchor the nucleus in position within the cell.
Nucleolus
The nucleolus is a dense region within the nucleus where ribosomal RNA (rRNA) biosynthesis occurs. In addition, the nucleolus is also the site where assembly of ribosomes begins. Preribosomal complexes are assembled from rRNA and proteins in the nucleolus; they are then transported out to the cytoplasm, where ribosome assembly is completed (Figure 5).
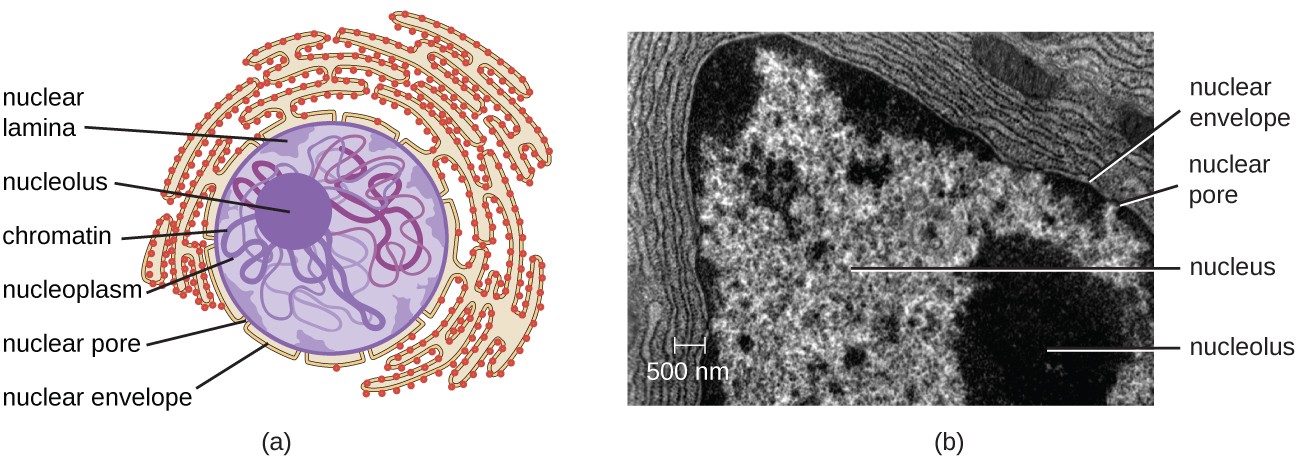
Figure 5. (a) The nucleolus is the dark, dense area within the nucleus. It is the site of rRNA synthesis and preribosomal assembly. (b) Electron micrograph showing the nucleolus.
Ribosomes
Ribosomes found in eukaryotic organelles such as mitochondria or chloroplasts have 70S ribosomes—the same size as prokaryotic ribosomes. However, nonorganelle-associated ribosomes in eukaryotic cells are 80S ribosomes, composed of a 40S small subunit and a 60S large subunit. In terms of size and composition, this makes them distinct from the ribosomes of prokaryotic cells.
The two types of nonorganelle-associated eukaryotic ribosomes are defined by their location in the cell: free ribosomes and membrane-bound ribosomes. Free ribosomes are found in the cytoplasm and serve to synthesize water-soluble proteins; membrane-bound ribosomes are found attached to the rough endoplasmic reticulum and make proteins for insertion into the cell membrane or proteins destined for export from the cell.
The differences between eukaryotic and prokaryotic ribosomes are clinically relevant because certain antibiotic drugs are designed to target one or the other. For example, cycloheximide targets eukaryotic action, whereas chloramphenicol targets prokaryotic ribosomes.[1] Since human cells are eukaryotic, they generally are not harmed by antibiotics that destroy the prokaryotic ribosomes in bacteria. However, sometimes negative side effects may occur because mitochondria in human cells contain prokaryotic ribosomes.
Endomembrane System
The endomembrane system, unique to eukaryotic cells, is a series of membranous tubules, sacs, and flattened disks that synthesize many cell components and move materials around within the cell (Figure 6). Because of their larger cell size, eukaryotic cells require this system to transport materials that cannot be dispersed by diffusion alone. The endomembrane system comprises several organelles and connections between them, including the endoplasmic reticulum, Golgi apparatus, lysosomes, and vesicles.
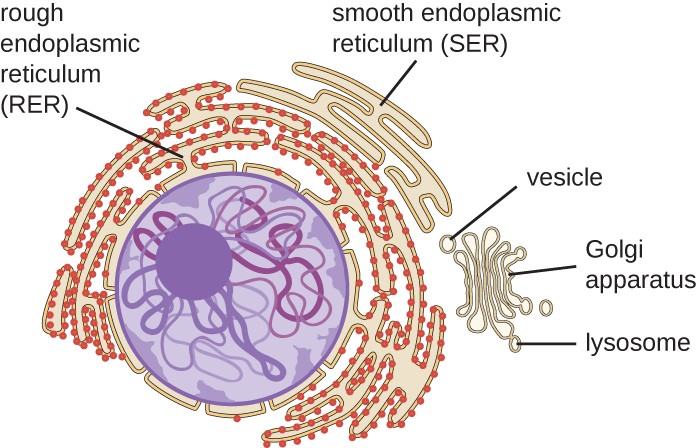
Figure 6. The endomembrane system is composed of a series of membranous intracellular structures that facilitate movement of materials throughout the cell and to the cell membrane.
Endoplasmic Reticulum
The endoplasmic reticulum (ER) is an interconnected array of tubules and cisternae (flattened sacs) with a single lipid bilayer (Figure 7). The spaces inside of the cisternae are called lumen of the ER. There are two types of ER, rough endoplasmic reticulum (RER) and smooth endoplasmic reticulum (SER). These two different types of ER are sites for the synthesis of distinctly different types of molecules. RER is studded with ribosomes bound on the cytoplasmic side of the membrane. These ribosomes make proteins destined for the plasma membrane (Figure 7). Following synthesis, these proteins are inserted into the membrane of the RER. Small sacs of the RER containing these newly synthesized proteins then bud off as transport vesicles and move either to the Golgi apparatus for further processing, directly to the plasma membrane, to the membrane of another organelle, or out of the cell. Transport vesicles are single-lipid, bilayer, membranous spheres with hollow interiors that carry molecules. SER does not have ribosomes and, therefore, appears “smooth.” It is involved in biosynthesis of lipids, carbohydrate metabolism, and detoxification of toxic compounds within the cell.
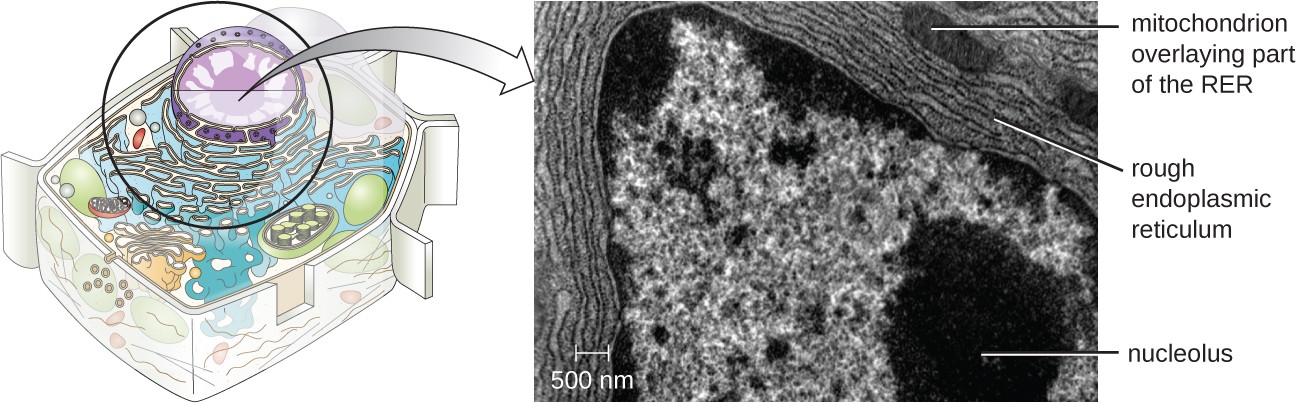
Figure 7. The rough endoplasmic reticulum is studded with ribosomes for the synthesis of membrane proteins (which give it its rough appearance).
Golgi Apparatus
The Golgi apparatus was discovered within the endomembrane system in 1898 by Italian scientist Camillo Golgi (1843–1926), who developed a novel staining technique that showed stacked membrane structures within the cells of Plasmodium, the causative agent of malaria. The Golgi apparatus is composed of a series of membranous disks called dictyosomes, each having a single lipid bilayer, that are stacked together (Figure 8).
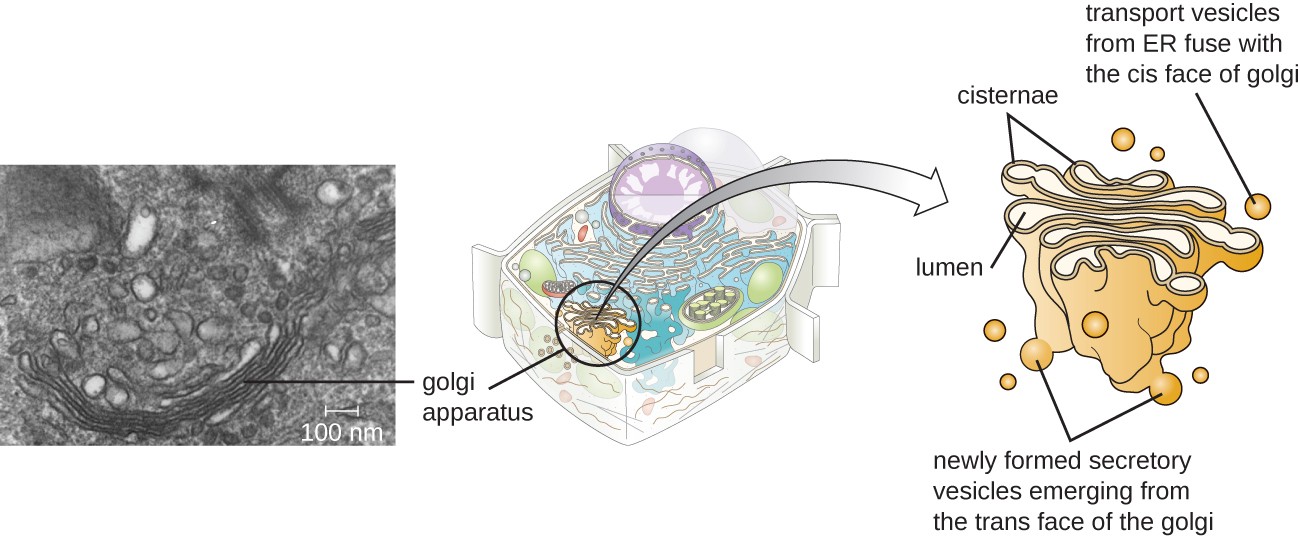
Figure 8. A transmission electron micrograph (left) of a Golgi apparatus in a white blood cell. The illustration (right) shows the cup-shaped, stacked disks and several transport vesicles. The Golgi apparatus modifies lipids and proteins, producing glycolipids and glycoproteins, respectively, which are commonly inserted into the plasma membrane.
Enzymes in the Golgi apparatus modify lipids and proteins transported from the ER to the Golgi, often adding carbohydrate components to them, producing glycolipids, glycoproteins, or proteoglycans. Glycolipids and glycoproteins are often inserted into the plasma membrane and are important for signal recognition by other cells or infectious particles. Different types of cells can be distinguished from one another by the structure and arrangement of the glycolipids and glycoproteins contained in their plasma membranes. These glycolipids and glycoproteins commonly also serve as cell surface receptors.
Transport vesicles leaving the ER fuse with a Golgi apparatus on its receiving, or cis, face. The proteins are processed within the Golgi apparatus, and then additional transport vesicles containing the modified proteins and lipids pinch off from the Golgi apparatus on its outgoing, or trans, face. These outgoing vesicles move to and fuse with the plasma membrane or the membrane of other organelles.
Exocytosis is the process by which secretory vesicles (spherical membranous sacs) release their contents to the cell’s exterior (Figure 8). All cells have constitutive secretory pathways in which secretory vesicles transport soluble proteins that are released from the cell continually (constitutively). Certain specialized cells also have regulated secretory pathways, which are used to store soluble proteins in secretory vesicles. Regulated secretion involves substances that are only released in response to certain events or signals. For example, certain cells of the human immune system (e.g., mast cells) secrete histamine in response to the presence of foreign objects or pathogens in the body. Histamine is a compound that triggers various mechanisms used by the immune system to eliminate pathogens.
Lysosomes
In the 1960s, Belgian scientist Christian de Duve (1917–2013) discovered lysosomes, membrane-bound organelles of the endomembrane system that contain digestive enzymes. Certain types of eukaryotic cells use lysosomes to break down various particles, such as food, damaged organelles or cellular debris, microorganisms, or immune complexes. Compartmentalization of the digestive enzymes within the lysosome allows the cell to efficiently digest matter without harming the cytoplasmic components of the cell.
Think about It
- Name the components of the endomembrane system and describe the function of each component.
Peroxisomes
Christian de Duve is also credited with the discovery of peroxisomes, membrane-bound organelles that are not part of the endomembrane system (Figure 9). Peroxisomes form independently in the cytoplasm from the synthesis of peroxin proteins by free ribosomes and the incorporation of these peroxin proteins into existing peroxisomes. Growing peroxisomes then divide by a process similar to binary fission.
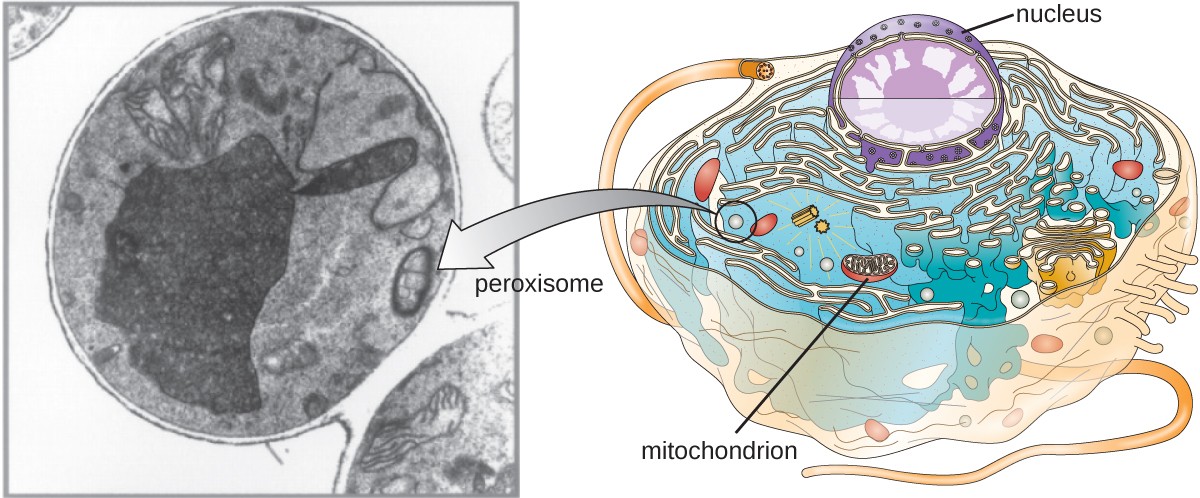
Figure 9. A transmission electron micrograph (left) of a cell containing a peroxisome. The illustration (right) shows the location of peroxisomes in a cell. These eukaryotic structures play a role in lipid biosynthesis and breaking down various molecules. They may also have other specialized functions depending on the cell type. (credit “micrograph”: modification of work by American Society for Microbiology)
Peroxisomes were first named for their ability to produce hydrogen peroxide, a highly reactive molecule that helps to break down molecules such as uric acid, amino acids, and fatty acids. Peroxisomes also possess the enzyme catalase, which can degrade hydrogen peroxide. Along with the SER, peroxisomes also play a role in lipid biosynthesis. Like lysosomes, the compartmentalization of these degradative molecules within an organelle helps protect the cytoplasmic contents from unwanted damage.
The peroxisomes of certain organisms are specialized to meet their particular functional needs. For example, glyoxysomes are modified peroxisomes of yeasts and plant cells that perform several metabolic functions, including the production of sugar molecules. Similarly, glycosomes are modified peroxisomes made by certain trypanosomes, the pathogenic protozoans that cause Chagas disease and African sleeping sickness.
Cytoskeleton
Eukaryotic cells have an internal cytoskeleton made of microfilaments, intermediate filaments, and microtubules. This matrix of fibers and tubes provides structural support as well as a network over which materials can be transported within the cell and on which organelles can be anchored (Figure 10). For example, the process of exocytosis involves the movement of a vesicle via the cytoskeletal network to the plasma membrane, where it can release its contents.
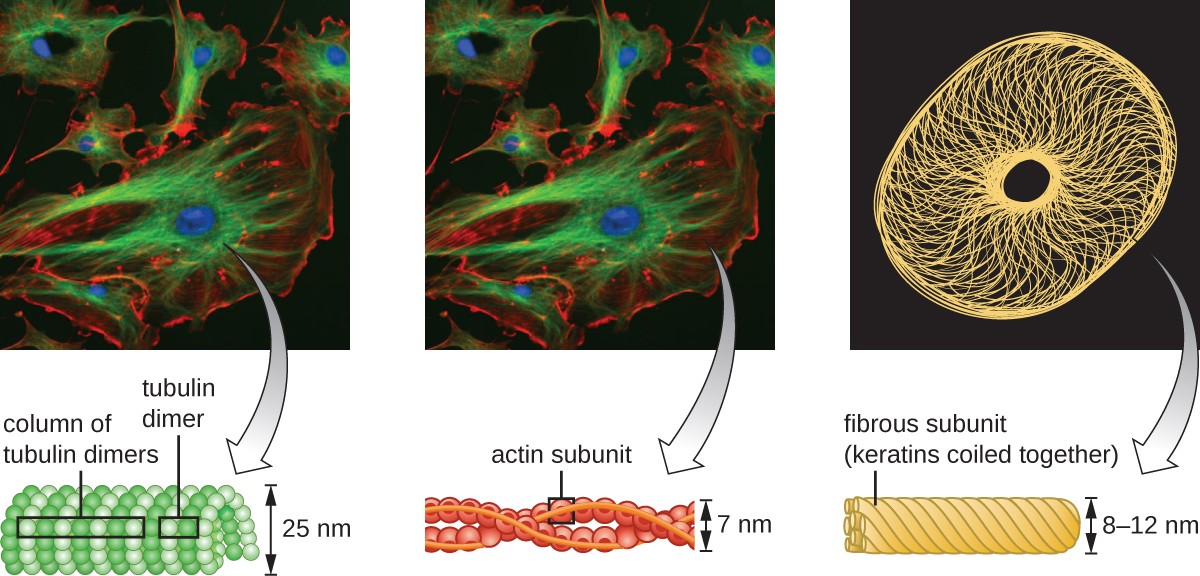
Figure 10. The cytoskeleton is a network of microfilaments, intermediate filaments, and microtubules found throughout the cytoplasm of a eukaryotic cell. In these fluorescently labeled animal cells, the microtubules are green, the actin microfilaments are red, the nucleus is blue, and keratin (a type of intermediate filament) is yellow.
Microfilaments are composed of two intertwined strands of actin, each composed of actin monomers forming filamentous cables 6 nm in diameter[2] (Figure 11). The actin filaments work together with motor proteins, like myosin, to effect muscle contraction in animals or the amoeboid movement of some eukaryotic microbes. In ameboid organisms, actin can be found in two forms: a stiffer, polymerized, gel form and a more fluid, unpolymerized soluble form. Actin in the gel form creates stability in the ectoplasm, the gel-like area of cytoplasm just inside the plasma membrane of ameboid protozoans.
Temporary extensions of the cytoplasmic membrane called pseudopodia (meaning “false feet”) are produced through the forward flow of soluble actin filaments into the pseudopodia, followed by the gel-sol cycling of the actin filaments, resulting in cell motility. Once the cytoplasm extends outward, forming a pseudopodium, the remaining cytoplasm flows up to join the leading edge, thereby creating forward locomotion. Beyond amoeboid movement, microfilaments are also involved in a variety of other processes in eukaryotic cells, including cytoplasmic streaming (the movement or circulation of cytoplasm within the cell), cleavage furrow formation during cell division, and muscle movement in animals (Figure 11). These functions are the result of the dynamic nature of microfilaments, which can polymerize and depolymerize relatively easily in response to cellular signals, and their interactions with molecular motors in different types of eukaryotic cells.
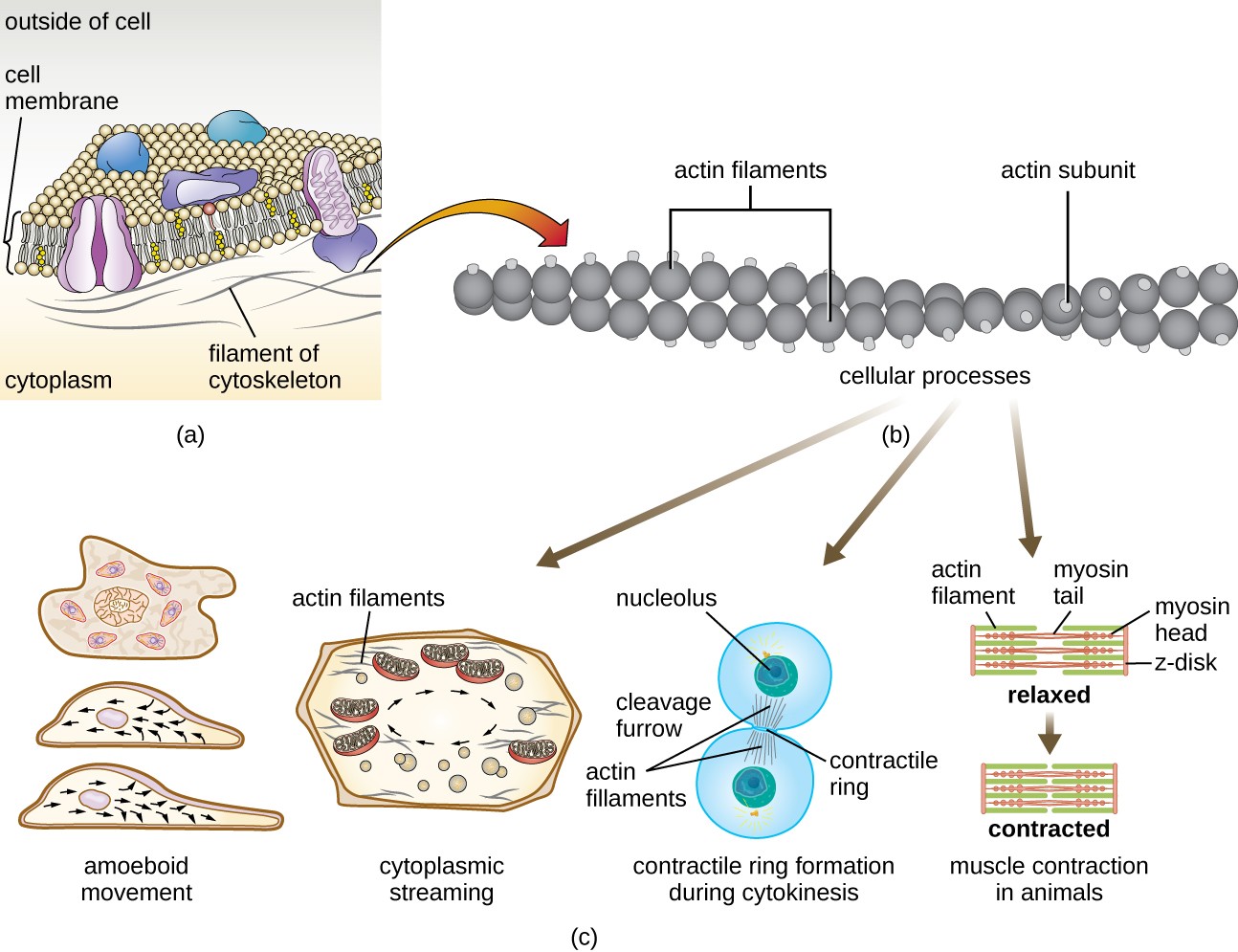
Figure 11. (a) A microfilament is composed of a pair of actin filaments. (b) Each actin filament is a string of polymerized actin monomers. (c) The dynamic nature of actin, due to its polymerization and depolymerization and its association with myosin, allows microfilaments to be involved in a variety of cellular processes, including ameboid movement, cytoplasmic streaming, contractile ring formation during cell division, and muscle contraction in animals.
Intermediate filaments (Figure 12) are a diverse group of cytoskeletal filaments that act as cables within the cell. They are termed “intermediate” because their 10-nm diameter is thicker than that of actin but thinner than that of microtubules.[3] They are composed of several strands of polymerized subunits that, in turn, are made up of a wide variety of monomers. Intermediate filaments tend to be more permanent in the cell and maintain the position of the nucleus. They also form the nuclear lamina (lining or layer) just inside the nuclear envelope. Additionally, intermediate filaments play a role in anchoring cells together in animal tissues. The intermediate filament protein desmin is found in desmosomes, the protein structures that join muscle cells together and help them resist external physical forces. The intermediate filament protein keratin is a structural protein found in hair, skin, and nails.
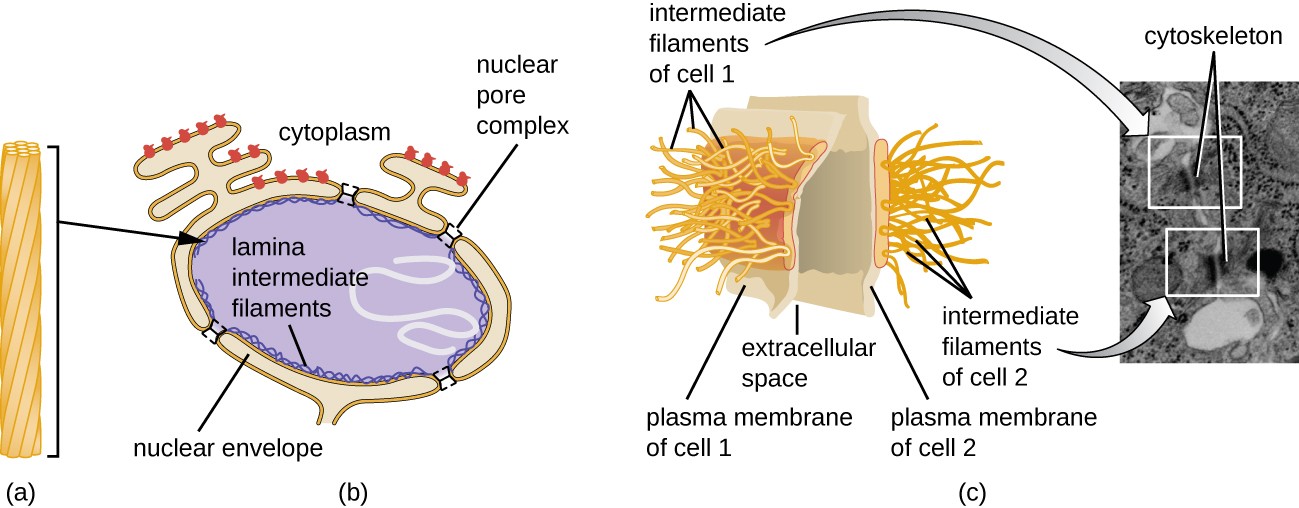
Figure 12. (a) Intermediate filaments are composed of multiple strands of polymerized subunits. They are more permanent than other cytoskeletal structures and serve a variety of functions. (b) Intermediate filaments form much of the nuclear lamina. (c) Intermediate filaments form the desmosomes between cells in some animal tissues. (credit c “illustration”: modification of work by Mariana Ruiz Villareal)
Microtubules (Figure 13) are a third type of cytoskeletal fiber composed of tubulin dimers (α tubulin and β tubulin). These form hollow tubes 23 nm in diameter that are used as girders within the cytoskeleton.[4] Like microfilaments, microtubules are dynamic and have the ability to rapidly assemble and disassemble. Microtubules also work with motor proteins (such as dynein and kinesin) to move organelles and vesicles around within the cytoplasm. Additionally, microtubules are the main components of eukaryotic flagella and cilia, composing both the filament and the basal body components (Figure 20).
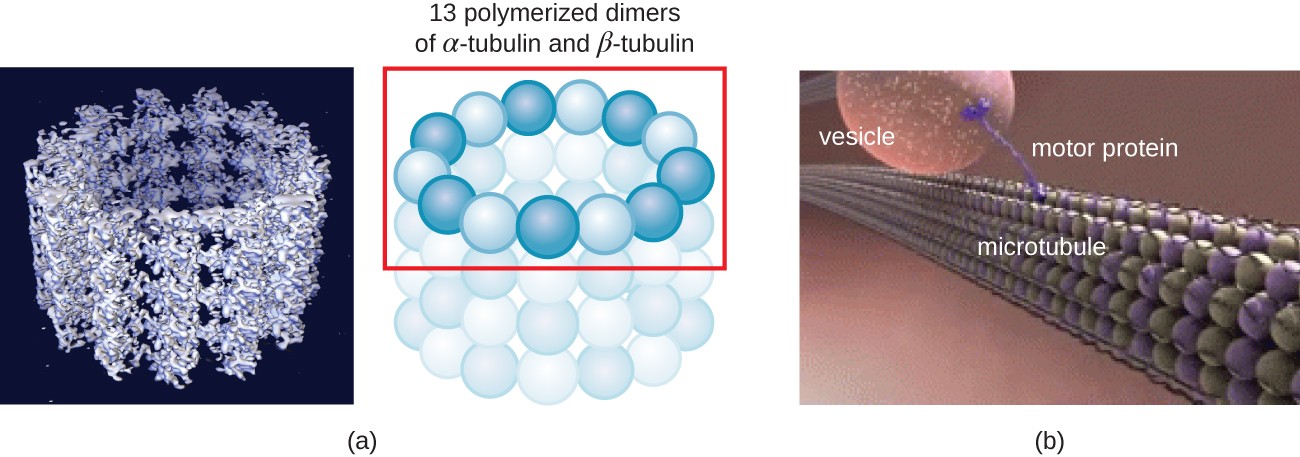
Figure 13. (a) Microtubules are hollow structures composed of polymerized tubulin dimers. (b) They are involved in several cellular processes, including the movement of organelles throughout the cytoplasm. Motor proteins carry organelles along microtubule tracks that crisscross the entire cell. (credit b: modification of work by National Institute on Aging)
In addition, microtubules are involved in cell division, forming the mitotic spindle that serves to separate chromosomes during mitosis and meiosis. The mitotic spindle is produced by two centrosomes, which are essentially microtubule-organizing centers, at opposite ends of the cell. Each centrosome is composed of a pair of centrioles positioned at right angles to each other, and each centriole is an array of nine parallel microtubules arranged in triplets (Figure 14).
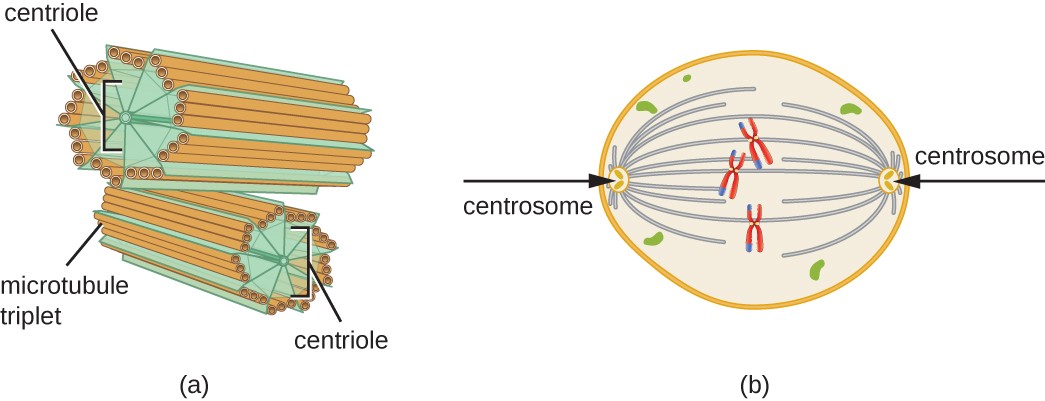
Figure 14. (a) A centrosome is composed of two centrioles positioned at right angles to each other. Each centriole is composed of nine triplets of microtubules held together by accessory proteins. (b) In animal cells, the centrosomes (arrows) serve as microtubule-organizing centers of the mitotic spindle during mitosis.
Think about It
- Compare and contrast the three types of cytoskeletal structures described in this section.
Mitochondria
The large, complex organelles in which aerobic cellular respiration occurs in eukaryotic cells are called mitochondria (Figure 15). The term “mitochondrion” was first coined by German microbiologist Carl Benda in 1898 and was later connected with the process of respiration by Otto Warburg in 1913. Scientists during the 1960s discovered that mitochondria have their own genome and 70S ribosomes. The mitochondrial genome was found to be bacterial, when it was sequenced in 1976. These findings ultimately supported the endosymbiotic theory proposed by Lynn Margulis, which states that mitochondria originally arose through an endosymbiotic event in which a bacterium capable of aerobic cellular respiration was taken up by phagocytosis into a host cell and remained as a viable intracellular component.
Each mitochondrion has two lipid membranes. The outer membrane is a remnant of the original host cell’s membrane structures. The inner membrane was derived from the bacterial plasma membrane. The electron transport chain for aerobic respiration uses integral proteins embedded in the inner membrane. The mitochondrial matrix, corresponding to the location of the original bacterium’s cytoplasm, is the current location of many metabolic enzymes. It also contains mitochondrial DNA and 70S ribosomes. Invaginations of the inner membrane, called cristae, evolved to increase surface area for the location of biochemical reactions. The folding patterns of the cristae differ among various types of eukaryotic cells and are used to distinguish different eukaryotic organisms from each other.
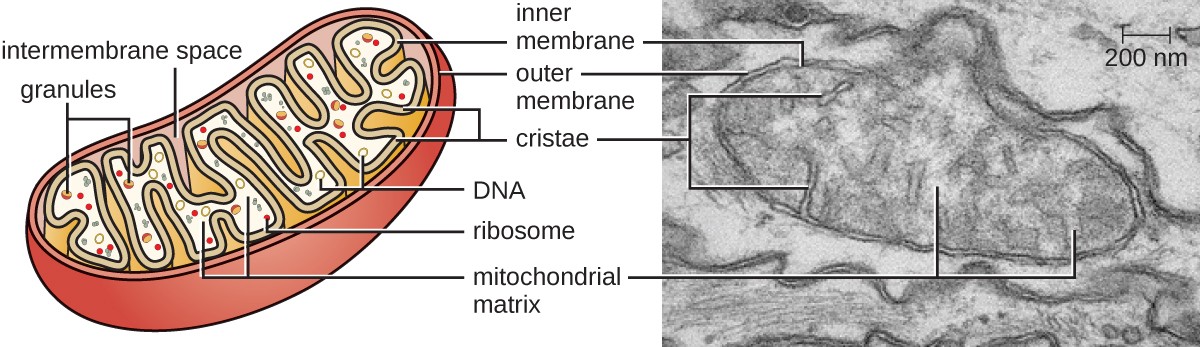
Figure 15. Each mitochondrion is surrounded by two membranes, the inner of which is extensively folded into cristae and is the site of the intermembrane space. The mitochondrial matrix contains the mitochondrial DNA, ribosomes, and metabolic enzymes. The transmission electron micrograph of a mitochondrion, on the right, shows both membranes, including cristae and the mitochondrial matrix. (credit “micrograph”: modification of work by Matthew Britton; scale-bar data from Matt Russell)
Chloroplasts
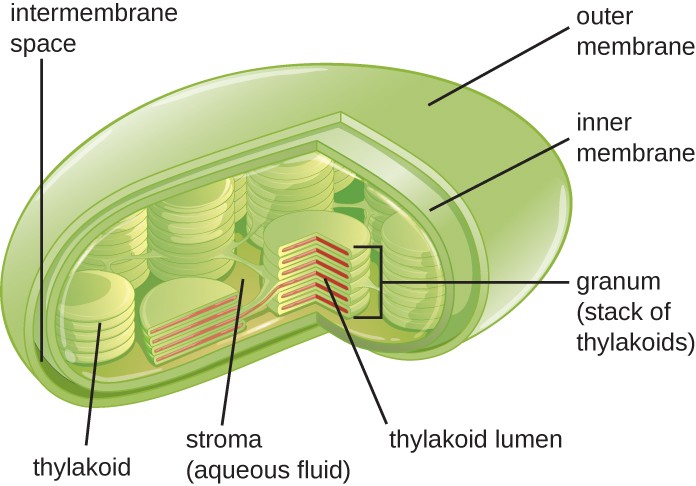
Figure 16. Photosynthesis takes place in chloroplasts, which have an outer membrane and an inner membrane. Stacks of thylakoids called grana form a third membrane layer.
Plant cells and algal cells contain chloroplasts, the organelles in which photosynthesis occurs (Figure 16). All chloroplasts have at least three membrane systems: the outer membrane, the inner membrane, and the thylakoid membrane system. Inside the outer and inner membranes is the chloroplast stroma, a gel-like fluid that makes up much of a chloroplast’s volume, and in which the thylakoid system floats. The thylakoid system is a highly dynamic collection of folded membrane sacs. It is where the green photosynthetic pigment chlorophyll is found and the light reactions of photosynthesis occur. In most plant chloroplasts, the thylakoids are arranged in stacks called grana (singular: granum), whereas in some algal chloroplasts, the thylakoids are free floating.
Other organelles similar to mitochondria have arisen in other types of eukaryotes, but their roles differ. Hydrogenosomes are found in some anaerobic eukaryotes and serve as the location of anaerobic hydrogen production. Hydrogenosomes typically lack their own DNA and ribosomes. Kinetoplasts are a variation of the mitochondria found in some eukaryotic pathogens. In these organisms, each cell has a single, long, branched mitochondrion in which kinetoplast DNA, organized as multiple circular pieces of DNA, is found concentrated at one pole of the cell.
Mitochondria-Related Organelles in Protozoan Parasites
Many protozoans, including several protozoan parasites that cause infections in humans, can be identified by their unusual appearance. Distinguishing features may include complex cell morphologies, the presence of unique organelles, or the absence of common organelles. The protozoan parasites Giardia lamblia and Trichomonas vaginalis are two examples.
G. lamblia, a frequent cause of diarrhea in humans and many other animals, is an anaerobic parasite that possesses two nuclei and several flagella. Its Golgi apparatus and endoplasmic reticulum are greatly reduced, and it lacks mitochondria completely. However, it does have organelles known as mitosomes, double-membrane-bound organelles that appear to be severely reduced mitochondria. This has led scientists to believe that G. lamblia’s ancestors once possessed mitochondria that evolved to become mitosomes. T. vaginalis, which causes the sexually transmitted infection vaginitis, is another protozoan parasite that lacks conventional mitochondria. Instead, it possesses hydrogenosomes, mitochondrial-related, double-membrane-bound organelles that produce molecular hydrogen used in cellular metabolism. Scientists believe that hydrogenosomes, like mitosomes, also evolved from mitochondria.[5]
Plasma Membrane
The plasma membrane of eukaryotic cells is similar in structure to the prokaryotic plasma membrane in that it is composed mainly of phospholipids forming a bilayer with embedded peripheral and integral proteins (Figure 17). These membrane components move within the plane of the membrane according to the fluid mosaic model. However, unlike the prokaryotic membrane, eukaryotic membranes contain sterols, including cholesterol, that alter membrane fluidity. Additionally, many eukaryotic cells contain some specialized lipids, including sphingolipids, which are thought to play a role in maintaining membrane stability as well as being involved in signal transduction pathways and cell-to-cell communication.
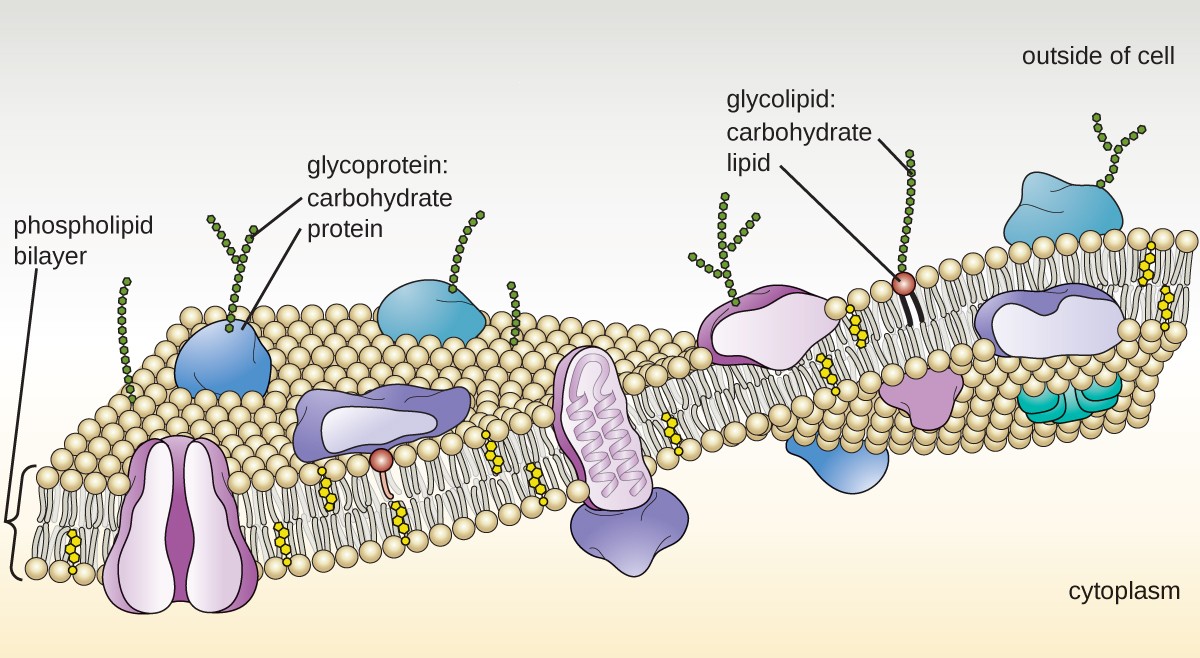
Figure 17. The eukaryotic plasma membrane is composed of a lipid bilayer with many embedded or associated proteins. It contains cholesterol for the maintenance of membrane, as well as glycoproteins and glycolipids that are important in the recognition other cells or pathogens.
Membrane Transport Mechanisms
The processes of simple diffusion, facilitated diffusion, and active transport are used in both eukaryotic and prokaryotic cells. However, eukaryotic cells also have the unique ability to perform various types of endocytosis, the uptake of matter through plasma membrane invagination and vacuole/vesicle formation (Figure 18). A type of endocytosis involving the engulfment of large particles through membrane invagination is called phagocytosis, which means “cell eating.” In phagocytosis, particles (or other cells) are enclosed in a pocket within the membrane, which then pinches off from the membrane to form a vacuole that completely surrounds the particle. Another type of endocytosis is called pinocytosis, which means “cell drinking.” In pinocytosis, small, dissolved materials and liquids are taken into the cell through small vesicles. Saprophytic fungi, for example, obtain their nutrients from dead and decaying matter largely through pinocytosis.
Receptor-mediated endocytosis is a type of endocytosis that is initiated by specific molecules called ligands when they bind to cell surface receptors on the membrane. Receptor-mediated endocytosis is the mechanism that peptide and amine-derived hormones use to enter cells and is also used by various viruses and bacteria for entry into host cells.
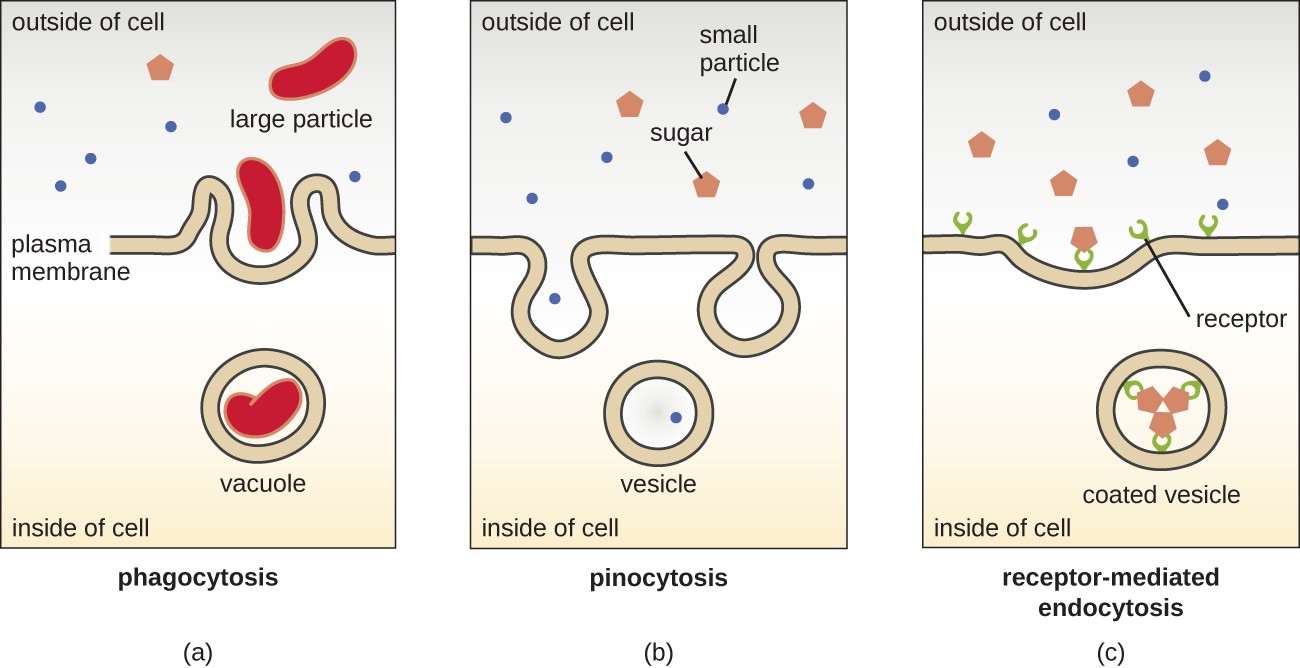
Figure 18. Three variations of endocytosis are shown. (a) In phagocytosis, the cell membrane surrounds the particle and pinches off to form an intracellular vacuole. (b) In pinocytosis, the cell membrane surrounds a small volume of fluid and pinches off, forming a vesicle. (c) In receptor-mediated endocytosis, the uptake of substances is targeted to a specific substance (a ligand) that binds at the receptor on the external cell membrane. (credit: modification of work by Mariana Ruiz Villarreal)
The process by which secretory vesicles release their contents to the cell’s exterior is called exocytosis. Vesicles move toward the plasma membrane and then meld with the membrane, ejecting their contents out of the cell. Exocytosis is used by cells to remove waste products and may also be used to release chemical signals that can be taken up by other cells.
Cell Wall
In addition to a plasma membrane, some eukaryotic cells have a cell wall. Cells of fungi, algae, plants, and even some protists have cell walls. Depending upon the type of eukaryotic cell, cell walls can be made of a wide range of materials, including cellulose (fungi and plants); biogenic silica, calcium carbonate, agar, and carrageenan (protists and algae); or chitin (fungi). In general, all cell walls provide structural stability for the cell and protection from environmental stresses such as desiccation, changes in osmotic pressure, and traumatic injury.[6]
Extracellular Matrix
Cells of animals and some protozoans do not have cell walls to help maintain shape and provide structural stability. Instead, these types of eukaryotic cells produce an extracellular matrix for this purpose. They secrete a sticky mass of carbohydrates and proteins into the spaces between adjacent cells (Figure 19). Some protein components assemble into a basement membrane to which the remaining extracellular matrix components adhere. Proteoglycans typically form the bulky mass of the extracellular matrix while fibrous proteins, like collagen, provide strength. Both proteoglycans and collagen are attached to fibronectin proteins, which, in turn, are attached to integrin proteins. These integrin proteins interact with transmembrane proteins in the plasma membranes of eukaryotic cells that lack cell walls.
In animal cells, the extracellular matrix allows cells within tissues to withstand external stresses and transmits signals from the outside of the cell to the inside. The amount of extracellular matrix is quite extensive in various types of connective tissues, and variations in the extracellular matrix can give different types of tissues their distinct properties. In addition, a host cell’s extracellular matrix is often the site where microbial pathogens attach themselves to establish infection. For example, Streptococcus pyogenes, the bacterium that causes strep throat and various other infections, binds to fibronectin in the extracellular matrix of the cells lining the oropharynx (upper region of the throat).
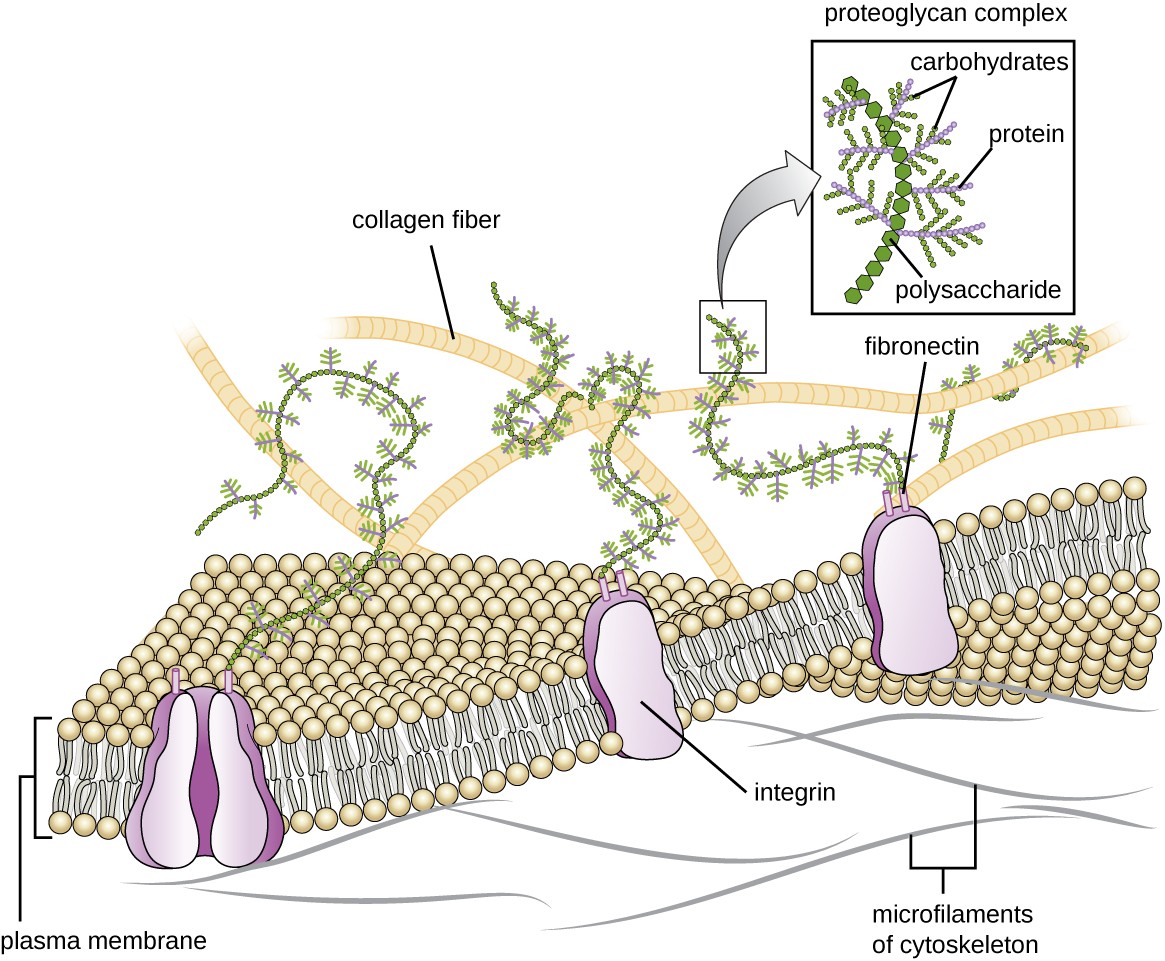
Figure 19. Click for a larger image. The extracellular matrix is composed of protein and carbohydrate components. It protects cells from physical stresses and transmits signals arriving at the outside edges of the tissue to cells deeper within the tissue.
Flagella and Cilia
Some eukaryotic cells use flagella for locomotion; however, eukaryotic flagella are structurally distinct from those found in prokaryotic cells. Whereas the prokaryotic flagellum is a stiff, rotating structure, a eukaryotic flagellum is more like a flexible whip composed of nine parallel pairs of microtubules surrounding a central pair of microtubules. This arrangement is referred to as a 9+2 array (Figure 20a). The parallel microtubules use dynein motor proteins to move relative to each other, causing the flagellum to bend.
Cilia (singular: cilium) are a similar external structure found in some eukaryotic cells. Unique to eukaryotes, cilia are shorter than flagella and often cover the entire surface of a cell; however, they are structurally similar to flagella (a 9+2 array of microtubules) and use the same mechanism for movement. A structure called a basal body is found at the base of each cilium and flagellum. The basal body, which attaches the cilium or flagellum to the cell, is composed of an array of triplet microtubules similar to that of a centriole but embedded in the plasma membrane. Because of their shorter length, cilia use a rapid, flexible, waving motion. In addition to motility, cilia may have other functions such as sweeping particles past or into cells. For example, ciliated protozoans use the sweeping of cilia to move food particles into their mouthparts, and ciliated cells in the mammalian respiratory tract beat in synchrony to sweep mucus and debris up and out of the lungs (Figure 20).
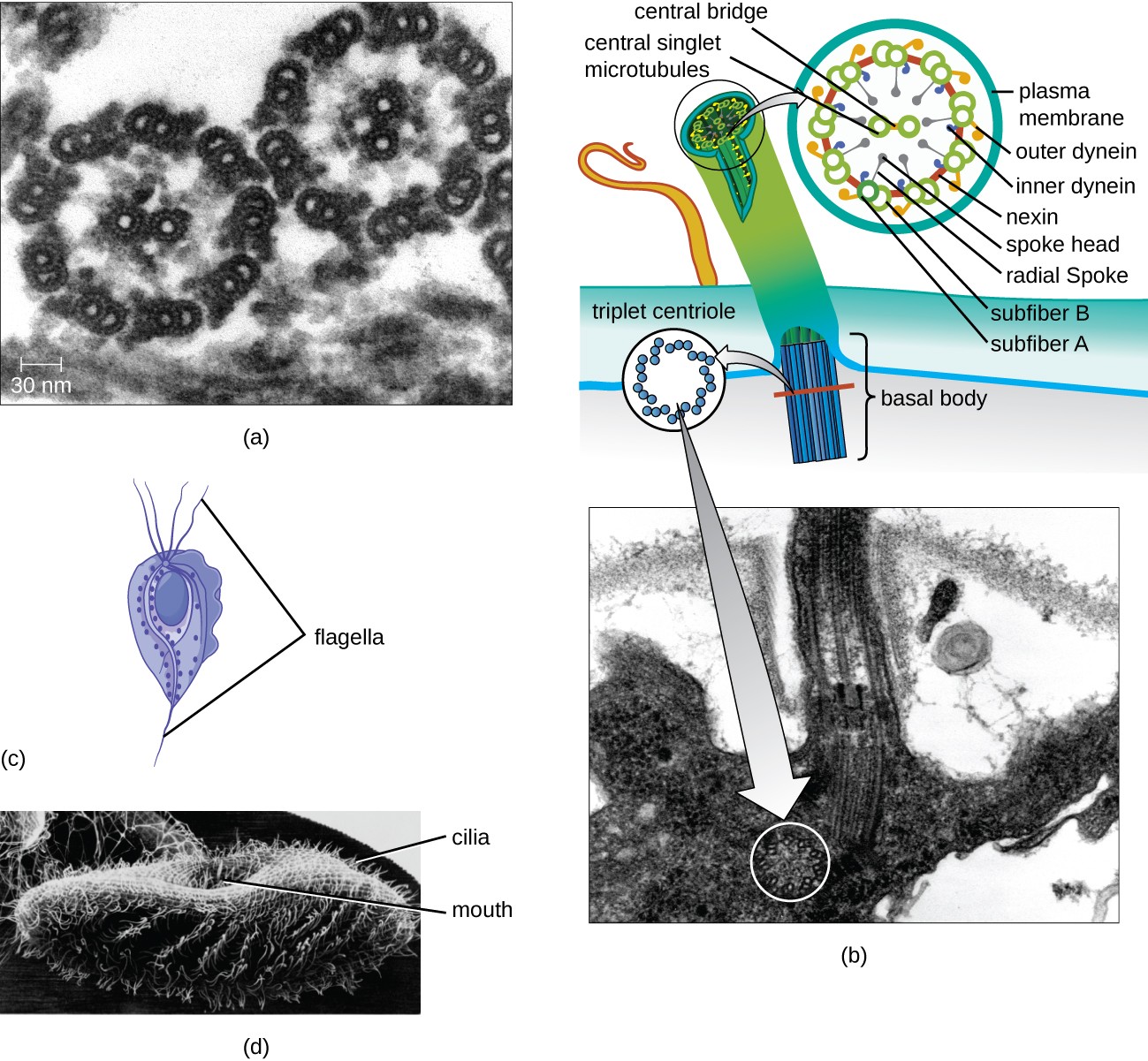
Figure 20. Click to view a larger image. (a) Eukaryotic flagella and cilia are composed of a 9+2 array of microtubules, as seen in this transmission electron micrograph cross-section. (b) The sliding of these microtubules relative to each other causes a flagellum to bend. (c) An illustration of Trichomonas vaginalis, a flagellated protozoan parasite that causes vaginitis. (d) Many protozoans, like this Paramecium, have numerous cilia that aid in locomotion as well as in feeding. Note the mouth opening shown here. (credit d: modification of work by University of Vermont/National Institutes of Health)
Think about It
- Explain how the cellular envelope of eukaryotic cells compares to that of prokaryotic cells.
- Explain the difference between eukaryotic and prokaryotic flagella.
Key Concepts and Summary
- Eukaryotic cells are defined by the presence of a nucleus containing the DNA genome and bound by a nuclear membrane (or nuclear envelope) composed of two lipid bilayers that regulate transport of materials into and out of the nucleus through nuclear pores.
- Eukaryotic cell morphologies vary greatly and may be maintained by various structures, including the cytoskeleton, the cell membrane, and/or the cell wall
- The nucleolus, located in the nucleus of eukaryotic cells, is the site of ribosomal synthesis and the first stages of ribosome assembly.
- Eukaryotic cells contain 80S ribosomes in the rough endoplasmic reticulum (membrane bound-ribosomes) and cytoplasm (free ribosomes). They contain 70s ribosomes in mitochondria and chloroplasts.
- Eukaryotic cells have evolved an endomembrane system, containing membrane-bound organelles involved in transport. These include vesicles, the endoplasmic reticulum, and the Golgi apparatus.
- The smooth endoplasmic reticulum plays a role in lipid biosynthesis, carbohydrate metabolism, and detoxification of toxic compounds. The rough endoplasmic reticulum contains membrane-bound 80S ribosomes that synthesize proteins destined for the cell membrane
- The Golgi apparatus processes proteins and lipids, typically through the addition of sugar molecules, producing glycoproteins or glycolipids, components of the plasma membrane that are used in cell-to-cell communication.
- Lysosomes contain digestive enzymes that break down small particles ingested by endocytosis, large particles or cells ingested by phagocytosis, and damaged intracellular components.
- The cytoskeleton, composed of microfilaments, intermediate filaments, and microtubules, provides structural support in eukaryotic cells and serves as a network for transport of intracellular materials.
- Centrosomes are microtubule-organizing centers important in the formation of the mitotic spindle in mitosis.
- Mitochondria are the site of cellular respiration. They have two membranes: an outer membrane and an inner membrane with cristae. The mitochondrial matrix, within the inner membrane, contains the mitochondrial DNA, 70S ribosomes, and metabolic enzymes.
- The plasma membrane of eukaryotic cells is structurally similar to that found in prokaryotic cells, and membrane components move according to the fluid mosaic model. However, eukaryotic membranes contain sterols, which alter membrane fluidity, as well as glycoproteins and glycolipids, which help the cell recognize other cells and infectious particles.
- In addition to active transport and passive transport, eukaryotic cell membranes can take material into the cell via endocytosis, or expel matter from the cell via exocytosis.
- Cells of fungi, algae, plants, and some protists have a cell wall, whereas cells of animals and some protozoans have a sticky extracellular matrix that provides structural support and mediates cellular signaling.
- Eukaryotic flagella are structurally distinct from prokaryotic flagella but serve a similar purpose (locomotion). Cilia are structurally similar to eukaryotic flagella, but shorter; they may be used for locomotion, feeding, or movement of extracellular particles.
Multiple Choice
Which of the following organelles is not part of the endomembrane system?
- endoplasmic reticulum
- Golgi apparatus
- lysosome
- peroxisome
Which type of cytoskeletal fiber is important in the formation of the nuclear lamina?
- microfilaments
- intermediate filaments
- microtubules
- fibronectin
Sugar groups may be added to proteins in which of the following?
- smooth endoplasmic reticulum
- rough endoplasmic reticulum
- Golgi apparatus
- lysosome
Which of the following structures of a eukaryotic cell is not likely derived from endosymbiotic bacterium?
- mitochondrial DNA
- mitochondrial ribosomes
- inner membrane
- outer membrane
Which type of nutrient uptake involves the engulfment of small dissolved molecules into vesicles?
- active transport
- pinocytosis
- receptor-mediated endocytosis
- facilitated diffusion
Which of the following is not composed of microtubules?
- desmosomes
- centrioles
- eukaryotic flagella
- eukaryotic cilia
True/False
Mitochondria in eukaryotic cells contain ribosomes that are structurally similar to those found in prokaryotic cells.
Fill in the Blank
Peroxisomes typically produce _____________, a harsh chemical that helps break down molecules.
Microfilaments are composed of _____________ monomers.
Think about It
- What existing evidence supports the theory that mitochondria are of prokaryotic origin?
- Why do eukaryotic cells require an endomembrane system?
- Name at least two ways that prokaryotic flagella are different from eukaryotic flagella.
- How are peroxisomes more like mitochondria than like the membrane-bound organelles of the endomembrane system? How do they differ from mitochondria?
- Why must the functions of both lysosomes and peroxisomes be compartmentalized?
- Label the lettered parts of this eukaryotic cell.
Candela Citations
- OpenStax Microbiology. Provided by: OpenStax CNX. Located at: http://cnx.org/contents/e42bd376-624b-4c0f-972f-e0c57998e765@4.2. License: CC BY: Attribution. License Terms: Download for free at http://cnx.org/contents/e42bd376-624b-4c0f-972f-e0c57998e765@4.2
- A.E. Barnhill, M.T. Brewer, S.A. Carlson. "Adverse Effects of Antimicrobials via Predictable or Idiosyncratic Inhibition of Host Mitochondrial Components." Antimicrobial Agents and Chemotherapy 56 no. 8 (2012):4046–4051. ↵
- Fuchs E, Cleveland DW. "A Structural Scaffolding of Intermediate Filaments in Health and Disease." Science 279 no. 5350 (1998):514–519. ↵
- E. Fuchs, D.W. Cleveland. "A Structural Scaffolding of Intermediate Filaments in Health and Disease." Science 279 no. 5350 (1998):514–519. ↵
- E. Fuchs, D.W. Cleveland. "A Structural Scaffolding of Intermediate Filaments in Health and Disease." Science 279 no. 5350 (1998):514–519. ↵
- N. Yarlett, J.H.P. Hackstein. "Hydrogenosomes: One Organelle, Multiple Origins." BioScience 55 no. 8 (2005):657–658. ↵
- M. Dudzick. "Protists." OpenStax CNX. November 27, 2013. https://cnx.org/contents/f7048bb6-e462-459b-805c-ef291cf7049c@1 ↵