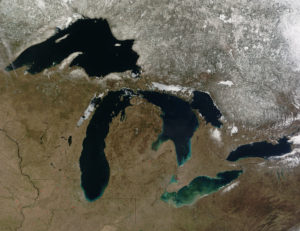
Great Lakes from Space. The Great Lakes hold 21% of the world’s surface fresh water. Lakes are an important surface water resource. Source: NASA Visible Earth
Introduction
Water, air, and food are the most important natural resources to people. Humans can live only a few minutes without oxygen, less than a week without water, and about a month without food. Water also is essential for our oxygen and food supply. Plants breakdown water and use it to create oxygen during the process of photosynthesis.
Water is the most essential compound for all living things. Human babies are approximately 75% water and adults are 60% water. Our brain is about 85% water, blood and kidneys are 83% water, muscles are 76% water, and even bones are 22% water. We constantly lose water by perspiration; in temperate climates we should drink about 2 quarts of water per day and people in hot desert climates should drink up to 10 quarts of water per day. Loss of 15% of body-water usually causes death.
Earth is truly the Water Planet. The abundance of liquid water on Earth’s surface distinguishes us from other bodies in the solar system. About 70% of Earth’s surface is covered by oceans and approximately half of Earth’s surface is obscured by clouds (also made of water) at any time. There is a very large volume of water on our planet, about 1.4 billion cubic kilometers (km3) (330 million cubic miles) or about 53 billion gallons per person on Earth. All of Earth’s water could cover the United States to a depth of 145 km (90 mi). From a human perspective, the problem is that over 97% of it is seawater, which is too salty to drink or use for irrigation. The most commonly used water sources are rivers and lakes, which contain less than 0.01% of the world’s water!
One of the most important environmental goals is to provide clean water to all people. Fortunately, water is a renewable resource and is difficult to destroy. Evaporation and precipitation combine to replenish our fresh water supply constantly; however, water availability is complicated by its uneven distribution over the Earth. Arid climate and densely populated areas have combined in many parts of the world to create water shortages, which are projected to worsen in the coming years due to population growth and climate change. Human activities such as water overuse and water pollution have compounded significantly the water crisis that exists today. Hundreds of millions of people lack access to safe drinking water, and billions of people lack access to improved sanitation as simple as a pit latrine. As a result, nearly two million people die every year from diarrheal diseases and 90% of those deaths occur among children under the age of 5. Most of these are easily prevented deaths.
Water Reservoirs and Water Cycle
Water is the only common substance that occurs naturally on earth in three forms: solid, liquid and gas. It is distributed in various locations, called water reservoirs. The oceans are by far the largest of the reservoirs with about 97% of all water but that water is too saline for most human uses (Figure 1). Ice caps and glaciers are the largest reservoirs of fresh water but this water is inconveniently located, mostly in Antarctica and Greenland. Shallow groundwater is the largest reservoir of usable fresh water. Although rivers and lakes are the most heavily used water resources, they represent only a tiny amount of the world’s water. If all of world’s water was shrunk to the size of 1 gallon, then the total amount of fresh water would be about 1/3 cup, and the amount of readily usable fresh water would be 2 tablespoons.
Figure 1. Earth’s Water Reservoirs. Bar chart Distribution of Earth’s water including total global water, fresh water, and surface water and other fresh water and Pie chart Water usable by humans and sources of usable water. Source: United States Geographical Survey Igor Skiklomanov’s chapter “World fresh water resources” in Peter H. Gleick (editor), 1993, Water in Crisis: A Guide to the World’s Fresh Water Resources
The water (or hydrologic) cycle (that was covered in Chapter 3.2) shows the movement of water through different reservoirs, which include oceans, atmosphere, glaciers, groundwater, lakes, rivers, and biosphere. Solar energy and gravity drive the motion of water in the water cycle. Simply put, the water cycle involves water moving from oceans, rivers, and lakes to the atmosphere by evaporation, forming clouds. From clouds, it falls as precipitation (rain and snow) on both water and land. The water on land can either return to the ocean by surface runoff, rivers, glaciers, and subsurface groundwater flow, or return to the atmosphere by evaporation or transpiration (loss of water by plants to the atmosphere).
Figure 2. The Water Cycle. Arrows depict movement of water to different reservoirs located above, at, and below Earth’s surface. Source: United States Geological Survey
An important part of the water cycle is how water varies in salinity, which is the abundance of dissolved ions in water. The saltwater in the oceans is highly saline, with about 35,000 mg of dissolved ions per liter of seawater. Evaporation (where water changes from liquid to gas at ambient temperatures) is a distillation process that produces nearly pure water with almost no dissolved ions. As water vaporizes, it leaves the dissolved ions in the original liquid phase. Eventually, condensation (where water changes from gas to liquid) forms clouds and sometimes precipitation (rain and snow). After rainwater falls onto land, it dissolves minerals in rock and soil, which increases its salinity. Most lakes, rivers, and near-surface groundwater have a relatively low salinity and are called freshwater. The next several sections discuss important parts of the water cycle relative to fresh water resources.
Primary Fresh Water Resources: Precipitation
Precipitation levels are unevenly distributed around the globe, affecting fresh water availability (Figure 3). More precipitation falls near the equator, whereas less precipitation tends to fall near 30 degrees north and south latitude, where the world’s largest deserts are located. These rainfall and climate patterns are related to global wind circulation cells. The intense sunlight at the equator heats air, causing it to rise and cool, which decreases the ability of the air mass to hold water vapor and results in frequent rainstorms. Around 30 degrees north and south latitude, descending air conditions produce warmer air, which increases its ability to hold water vapor and results in dry conditions. Both the dry air conditions and the warm temperatures of these latitude belts favor evaporation. Global precipitation and climate patterns are also affected by the size of continents, major ocean currents, and mountains.
Figure 3. World Rainfall Map. The false-color map above shows the amount of rain that falls around the world. Areas of high rainfall include Central and South America, western Africa, and Southeast Asia. Since these areas receive so much rainfall, they are where most of the world’s rainforests grow. Areas with very little rainfall usually turn into deserts. The desert areas include North Africa, the Middle East, western North America, and Central Asia. Source: United States Geological Survey Earth Forum, Houston Museum Natural Science
Surface Water Resources: Rivers, Lakes, Glaciers
Flowing water from rain and melted snow on land enters river channels by surface runoff (Figure 4) and groundwater seepage (Figure 5). River discharge describes the volume of water moving through a river channel over time (Figure 6). The relative contributions of surface runoff vs. groundwater seepage to river discharge depend on precipitation patterns, vegetation, topography, land use, and soil characteristics. Soon after a heavy rainstorm, river discharge increases due to surface runoff. The steady normal flow of river water is mainly from groundwater that discharges into the river. Gravity pulls river water downhill toward the ocean. Along the way the moving water of a river can erode soil particles and dissolve minerals. Groundwater also contributes a large amount of the dissolved minerals in river water. The geographic area drained by a river and its tributaries is called a drainage basin or watershed. The Mississippi River drainage basin includes approximately 40% of the U.S., a measure that includes the smaller drainage basins, such as the Ohio River and Missouri River that help to comprise it. Rivers are an important water resource for irrigation of cropland and drinking water for many cities around the world. Rivers that have had international disputes over water supply include the Colorado (Mexico, southwest U.S.), Nile (Egypt, Ethiopia, Sudan), Euphrates (Iraq, Syria, Turkey), Ganges (Bangladesh, India), and Jordan (Israel, Jordan, Syria).
Figure 4. Surface Runoff Surface runoff, part of overland flow in the water cycle Source: James M. Pease at Wikimedia Commons
Figure 5. Groundwater Seepage. Groundwater seepage can be seen in Box Canyon in Idaho, where approximately 10 cubic meters per second of seepage emanates from its vertical headwall. Source: NASA
Figure 6. River Discharge Colorado River, U.S.. Rivers are part of overland flow in the water cycle and an important surface water resource. Source: Gonzo fan2007 at Wikimedia Commons.
In addition to rivers, lakes can also be an excellent source of freshwater for human use. They usually receive water from surface runoff and groundwater discharge. They tend to be short-lived on a geological time-scale because they are constantly filling in with sediment supplied by rivers. Lakes form in a variety of ways including glaciation, recent tectonic uplift (e.g., Lake Tanganyika, Africa), and volcanic eruptions (e.g., Crater Lake, Oregon). People also create artificial lakes (reservoirs) by damming rivers. Large changes in climate can result in major changes in a lake’s size. As Earth was coming out of the last Ice Age about 15,000 years ago, the climate in the western U.S. changed from cool and moist to warm and arid, which caused more than 100 large lakes to disappear. The Great Salt Lake in Utah is a remnant of a much larger lake called Lake Bonneville.
Great Lakes from Space The Great Lakes hold 21% of the world’s surface fresh water. Lakes are an important surface water resource. Source: SeaWiFS Project, NASA/Goddard Space Flight Center, and ORBIMAGE
Although glaciers represent the largest reservoir of fresh water, they generally are not used as a water source because they are located too far from most people (Figure 7). Melting glaciers do provide a natural source of river water and groundwater. During the last Ice Age there was as much as 50% more water in glaciers than there is today, which caused sea level to be about 100 m lower. Over the past century, sea level has been rising in part due to melting glaciers. If Earth’s climate continues to warm, the melting glaciers will cause an additional rise in sea level.
Figure 7. Mountain Glacier in Argentina Glaciers are the largest reservoir of fresh water but they are not used much as a water resource directly by society because of their distance from most people. Source: Luca Galuzzi – www.galuzzi.it
Groundwater Resources
Although most people in the U.S. and the world use surface water, groundwater is a much larger reservoir of usable fresh water, containing more than 30 times more water than rivers and lakes combined. Groundwater is a particularly important resource in arid climates, where surface water may be scarce. In addition, groundwater is the primary water source for rural homeowners, providing 98% of that water demand in the U.S.. Groundwater is water located in small spaces, called pore space, between mineral grains and fractures in subsurface earth materials (rock or sediment, i.e., loose grains). Groundwater is not located in underground rivers or lakes except where there are caves, which are relatively rare. Between the land surface and the depth where there is groundwater is the unsaturated zone, where pore spaces contain only air and water films on mineral grains (see Figure Subsurface Water Terminology).1 Below the unsaturated zone is the saturated zone, where groundwater completely fills pore spaces in earth materials. The interface between the unsaturated zone and saturated zone is the water table. Most groundwater originates from rain or snowmelt, which infiltrates the ground and moves downward until it reaches the saturated zone. Other sources of groundwater include seepage from surface water (lakes, rivers, reservoirs, and swamps), surface water deliberately pumped into the ground, irrigation, and underground wastewater treatment systems, i.e., septic tanks. Recharge areas are locations where surface water infiltrates the ground rather than running off into rivers or evaporating. Wetlands and flat vegetated areas in general are excellent recharge areas.

Figure 8: Subsurface Water Terminology Groundwater in pore spaces and fractures of earth materials, saturated zone, unsaturated zone, and water table, which follows land surface but in a more subdued way. Source: United States Geological Survey
Groundwater is in constant motion due to interconnection between pore spaces. Porosity is the percentage of pore space in an earth material and it gives a measure of how much groundwater an earth material can hold. Permeability is a measure of the speed that groundwater can flow through an earth material, and it depends on the size and degree of interconnection among pores. An earth material that is capable of supplying groundwater from a well at a useful rate—i.e., it has relatively high permeability and medium to high porosity—is called an aquifer. Examples of aquifers are earth materials with abundant, large, well-connected pore spaces such as sand, gravel, uncemented sandstone, and any highly fractured rock. An earth material with low hydraulic conductivity is an aquitard. Examples of aquitards include clay, shale (sedimentary rock with abundant clay), and igneous and metamorphic rock, if they contain few fractures.
As discussed above, groundwater flows because most earth materials near the surface have finite (nonzero) porosity and permeability values. Another reason for groundwater movement is that the surface of the water table commonly is not completely flat but mimics the topography of the land surface, especially in humid climates. There is “topography” to the water table because groundwater moves slowly through rock and soil, so it builds up in higher elevation areas. In fact, when groundwater flows slowly through aquitards and deep underground, it can take many thousands of years to move relatively short distances. An unconfined aquifer has no aquitard above it and, therefore, it is exposed to the atmosphere and surface waters through interconnected pores (See Figure Flowing Groundwater). In an unconfined aquifer, groundwater flows because of gravity to lower water table levels, where it eventually may discharge or leave the groundwater flow system. Discharge areas include rivers, lakes, swamps, reservoirs, water wells, and springs (see Figure Fatzael Springs in Jordan Valley). Springs are rivers that emerge from underground due to an abrupt intersection of the land surface and the water table caused by joints, caves, or faults that bring permeable earth materials to the surface. A confined aquifer is bounded by aquitards below and above, which prevents recharge from the surface immediately above. Instead, the major recharge occurs where the confined aquifer intercepts the land surface, which may be a long distance from water wells and discharge areas (see Figure Schematic Cross Section of Aquifer Types). Confined aquifers are commonly inclined away from recharge areas, so groundwater in a confined aquifer is under greater-than-atmospheric pressure due to the weight of water in the upslope direction. Similar to river discharge, groundwater discharge describes the volume of water moving through an aquifer over time. Total groundwater discharge depends on the permeability of the earth material, the pressure that drives groundwater flow, and the size of the aquifer. It is important to determine groundwater discharge to evaluate whether an aquifer can meet the water needs of an area.



Most shallow water wells are drilled into unconfined aquifers. These are called water table wells because the water level in the well coincides with the water table (See Figure Schematic Cross Section of Aquifer Types). 90% of all aquifers for water supply are unconfined aquifers composed of sand or gravel. To produce water from a well, you simply need to drill a hole that reaches the saturated zone and then pump water to the surface. Attempting to pump water from the unsaturated zone is like drinking root beer with a straw immersed only in the foam at the top.
To find a large aquifer for a city, hydrogeologists (geologists who specialize in groundwater) use a variety of information including knowledge of earth materials at the surface and sub-surface as well as test wells. Some people search for water by dowsing, where someone holds a forked stick or wire (called a divining rod) while walking over an area. The stick supposedly rotates or deflects downward when the dowser passes over water. Controlled tests show that a dowser’s success is equal to or less than random chance. Nevertheless, in many areas water wells are still drilled on dowser’s advice sometimes for considerable money. There is no scientific basis to dowsing.
Wells into confined aquifers typically are deeper than those into unconfined aquifers because they must penetrate a confining layer. The water level in a well drilled into a confined aquifer, which is an artesian well, (see Figure Schematic Cross Section of Aquifer Types), moves above the local water table to a level called the potentiometric surface because of the greater pressure on the groundwater. Water in a flowing well (see Figure A Flowing Well) moves all of the way to the land surface without pumping.

A confined aquifer tends to be depleted from groundwater pumping more quickly than an unconfined aquifer, assuming similar aquifer properties and precipitation levels. This is because confined aquifers have smaller recharge areas, which may be far from the pumping well. Conversely, an unconfined aquifer tends to be more susceptible to pollution because it is hydrologically connected to the surface, which is the source of most pollution.
Groundwater and surface water (rivers, lakes, swamps, and reservoirs) are strongly interrelated because both are part of the same overall resource. Major groundwater removal (from pumping or drought) can lower the levels of surface water and vice versa. We can define two types of streams: gaining (effluent) streams and losing (influent) streams (see Figure Interaction of Streams and Ground Water). Gaining streams tend to be perennial (flow year round), are characteristic of humid climates, have the water table sloping towards the river, and therefore gain water from groundwater discharge. Losing streams tend to be ephemeral (flow only after significant rain), are characteristic of arid climates, are located above the water table (which slopes away from the river), and therefore lose water to groundwater recharge. Pollution that is dumped into a losing stream will tend to move into the ground and could also contaminate local groundwater.

Water Use in the U.S. and World
People need water to produce the food, energy, and mineral resources they use—commonly large amounts of it. Consider, for example, these approximate water requirements for some things people in the developed world use every day: one tomato = 3 gallons; one kilowatt-hour of electricity (from a thermoelectric power plant) = 21 gallons; one loaf of bread = 150 gallons; one pound of beef = 1,600 gallons; and one ton of steel = 63,000 gallons. Human beings require only about 1 gallon per day to survive, but a typical person in a U.S. household uses approximately 100 gallons per day, which includes cooking, washing dishes and clothes, flushing the toilet, and bathing.
The water demand of an area is a function of the population and other uses of water. There are several general categories of water use, including offstream use, which removes water from its source, e.g., irrigation, thermoelectric power generation (cooling electricity-producing equipment in fossil fuel, nuclear, and geothermal power plants), industry, and public supply; consumptive use, which is a type of offstream use where water does not return to the surface water or groundwater system immediately after use, e.g., irrigation water that evaporates or goes to plant growth; and instream use, which is water used but not removed from a river, mostly for hydroelectric power generation. The relative size of these three categories are instream use >> offstream use > consumptive use. In 2005, the U.S. used approximately 3,300 billion gallons per day for instream use, 410 billion gallons per day for offstream use, and 100 billion gallons per day for consumptive use. The major offstream uses of that water were thermoelectric (49%), irrigation (31%), public supply (11%), and industry (4%, see Figure Trends in Total Water Withdrawals by Water-use Category, 1950-2005). About 15% of the total water withdrawals in the U.S. in 2005 were saline water, which was used almost entirely for thermoelectric power generation. Almost all of the water used for thermoelectric power generation is returned to the river, lake, or ocean from where it came but about half of irrigation water does not return to the original source due to evaporation, plant transpiration, and loss during transport, e.g., leaking pipes. Total withdrawals of water in the U.S. actually decreased slightly from 1980 to 2005, despite a steadily increasing population. This is because the two largest categories of water use (thermoelectric and irrigation) stabilized or decreased over that time period due to better water management and conservation. In contrast, public supply water demand increased steadily from 1950 (when estimates began) through 2005. Approximately 77% of the water for offstream use in the U.S. in 2005 came from surface water and the rest was from groundwater (see Figure Trends in Source of Fresh Water Withdrawals in the U.S. from 1950 to 2005).
Figure 8. Trends in Total Water Withdrawals by Water-use Category, 1950-2005 Trends in total water withdrawals in the U.S. from 1950 to 2005 by water use category, including bars for thermoelectric power, irrigation, public water supply, and rural domestic and livestock. Thin blue line represents total water withdrawals using vertical scale on right. Source: United States Geological Survey
Figure 9. Trends in Source of Fresh Water Withdrawals in the U.S. from 1950 to 2005 Trends in source of fresh water withdrawals in the U.S. from 1950 to 2005, including bars for surface water, groundwater, and total water. Red line gives U.S. population using vertical scale on right. Source: United States Geological Survey
Global total water use is steadily increasing at a rate greater than world population growth (Figure 10). During the 20th century global population tripled and water demand grew by a factor of six. The increase in global water demand beyond the rate of population growth is due to improved standard of living without an offset by water conservation. Increased production of goods and energy entails a large increase in water demand. The major global water uses are irrigation (68%), public supply (21%), and industry (11%). In contrast to trends in the U.S., global total water use is steadily increasing at a rate greater than world population growth (see Figure Trends in World Water Use from 1900 to 2000 and Projected to 2025). During the twentieth century global population tripled and water demand grew by a factor of six. The increase in global water demand beyond the rate of population growth is due to improved standard of living without an offset by water conservation. Increased production of goods and energy entails a large increase in water demand. The major global offstream water uses are irrigation (68%), public supply (21%), and industry (11%).
Figure 10. Trends in World Water Use from 1900 to 2000 and Projected to 2025 For each water major use category, including trends for agriculture, domestic use, and industry. Darker colored bar represents total water extracted for that use category and lighter colored bar represents water consumed (i.e., water that is not quickly returned to surface water or groundwater system) for that use category. Source: Igor A. Shiklomanow, State Hydrological Institute (SHI, St. Petersburg) and United Nations Educational, Scientific and Cultural Organisation (UNESCO, Paris), 1999