Learning Objectives
In this module, the following topics are covered: 1) the content and the goals of four-step of risk assessment process, 2) how to estimate dose received via each exposure pathway, 3) how to integrate exposure and toxicity information to characterize health risks, 4) how to quantitatively estimate cumulative cancer and noncancer risks, and 5) how to identify/evaluate uncertainties in risk assessment.
After reading this module, students should be able to
- understand the content and the goals of four-step of risk assessment process
- know how to estimate dose received via each exposure pathway
- know how to integrate exposure and toxicity information to characterize health risks
- understand how to quantitatively estimate cumulative cancer and noncancer risks
- understand how to identify/evaluate uncertainties in risk assessment
Introduction
Risk assessment is a scientific process used by federal agencies and risk management decision-makers to make informed decisions about actions that may be taken to protect human health by ascertaining potential human health risks or health hazard associated with exposure to chemicals in the environment.
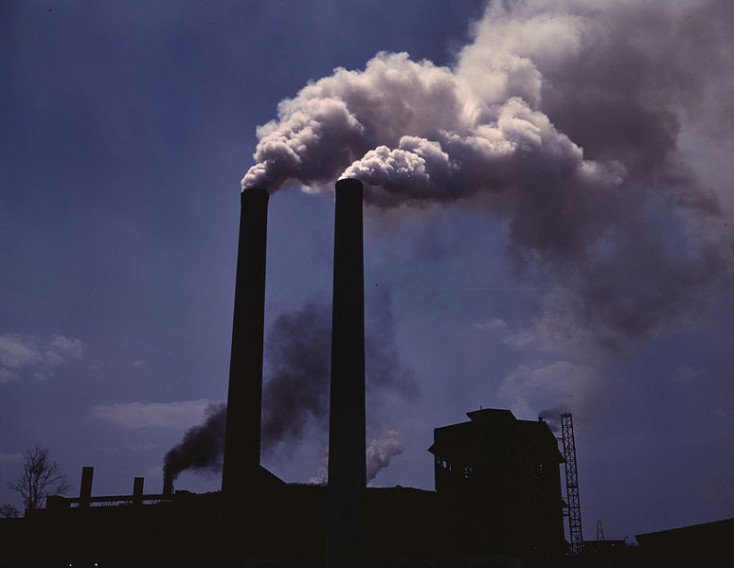
Smoke Stack Emissions into the Atmosphere Figure shows emissions billowing from a smoke stack into the atmosphere. Risk assessment helps federal agencies and risk management decision-makers arrive at informed decisions about actions to take to protect human health from hazards such as air pollution, pictured here. Source: Alfred Palmer via Wikipedia
Some of the real-world examples of risk assessment includes: establishment of national ambient air quality and drinking water standards for protection of public health (e.g. ozone, particulate matter in outdoor air; chromium, chloroform or benzene in water); establishment of clean-up levels for hazardous waste site remediation; development of fish consumption advisories for pregnant women and general population (e.g. PCBs, mercury); assessment of risks and benefits of different alternative fuels for sound energy policy development (e.g. oxygenated gasoline, biodiesel); and estimation of health risks associated with pesticide residues in food. The estimated risk is a function of exposure and toxicity, as described in detail in NAS (1983) and EPA (1989). The regulatory risk assessment follows a four-step paradigm using qualitative and/or quantitative approaches. In quantitative risk assessment using either deterministic or probabilistic approaches, the risk estimates pertaining to an exposure scenario is particularly useful when comparing a number of exposure or risk reduction measures among one another as an optimization protocol to determine the best economically viable option for protection of public health and the environment. With environmental sustainability and life-cycle analysis in the forefront of green technological innovation, energy, and economic savings in the 21st Century, risk assessment will pay a pivotal role in discerning the option(s) with the most benefit in health protection and, thus, will be an integral part of any environmentally sustainability analysis. Such comparative risk assessment can be performed for traditional approaches vs. environmentally sustainable approaches. They can also be performed among different environmentally sustainable options for an environmental pollution problem such hazardous waste site remediation and redevelopment, air quality management in urban areas, pest management practices, agricultural health and safety, alternative energy sources for transportation sources and among others.
The four steps of risk assessment are i) hazard identification; ii) toxicity (or dose-response) assessment; iii) exposure assessment; and iv) risk characterization, which are described below in detail. The emphasis is given in documenting the resources necessary to successfully perform each step.
Hazard Identification
In the hazard identification step, a scientific weight of evidence analysis is performed to determine whether a particular substance or chemical is or is not causally linked to any particular health effect at environmentally relevant concentrations. Hazard identification is performed to determine whether, and to what degree, toxic effects in one setting will occur in other settings. For example, is a chemical that is shown to cause carcinogenicity in animal test species (e.g. rat, mouse) likely to be a carcinogen in exposed humans? In order to assess the weight of evidence for adverse health effects, risk analysts follow the following steps (EPA, 1993): (1) Compile and analyze all the available toxicology data on the substance of interest; (2) Weigh the evidence that the substance causes a toxic effect (cancer of non-cancer health end-points); and (3) Assess whether adverse health effect (or toxicity) could occur from human exposure in a real-life setting.
In the first task of hazard identification, risk analyst examines the toxicity literature using the following analytical tools in the order of importance:
- Epidemiological studies
- Controlled human exposure chamber experiments
- In-vivo animal bioassays
- In-vitro cell and tissue culture bioassays
- Quantitative Structure –Activity Relationship Analysis (QSAR)
Among these, in-vivo animal bioassays are, by far, the most utilized source of information for hazard identification for chemicals and, on rare instances, for chemical mixtures (e.g. diesel). When available, well-conducted epidemiological studies are regarded as the most valuable source of human health hazard identification information since they provide direct human evidence for potential health effects. Epidemiology is the study of the occurrence and distribution of a disease or physiological condition in human populations and of the factors that influence this distribution (Lilienfeld and Lilienfeld, 1980). The advantages of epidemiological studies for hazard identification are (EPA, 1989; EPA, 1993): animal-to-human extrapolation is not necessary, real exposure conditions, and a wide range of subjects with different genetic and life-style patterns. However, epidemiological studies have a number of shortcomings, which limit their usefulness in hazard identification. Some of these disadvantages include difficulty in recruiting and maintaining a control group; having no control over some of the non-statistical variables related to exposures, lifestyles, co-exposure to other chemicals, etc.; absence of actual exposure measurements along with memory bias for retrospective studies; lengthy latency periods for chronic health effects such as cancer; and poor sensitivity and inability to determine cause-effect relationships conclusively.
Animal bioassays remedy some of the weaknesses of epidemiological studies by allowing for greater control over the experiment and are deemed to be reliable measurement of toxic effects, although they require “high dose in animals-to low dose in humans” extrapolation. The selection of design parameters of animal bioassays is critically important in observing or missing an actual hazard. These parameters include: animal species selected for the experiment (rat, mouse); strain of the test species; age/sex of the test species; magnitude of exposure concentrations/doses applied or administered; number of dose levels studied; duration of exposure; controls selected; and route of exposure. Animal studies are characterized as acute (a single dose or exposures of short duration), chronic (exposures for full lifetimes of test species – about two years in rats/mice) and sub-chronic (usually 90 days) based on the exposure duration. In the hazard identification step, the following measures of toxicity are commonly compiled:
- LD50/LC50/EC50: The dose or concentration in a toxicity study at which causing 50 percent mortality in test species was observed. The EC50 is the effective concentration causing adverse effects or impairment in 50% of the test species.
- NOAEL (No Observable Adverse Effect Level): The highest dose or concentration in a toxicity study at which no adverse effect was observed.
- LOAEL (Lowest Observable Adverse Effect Level): The lowest dose or concentration in a toxicity study at which an adverse effect was observed.
- MTD (Maximum Tolerated Dose): The largest dose a test animal can receive for most of its lifetime without demonstrating adverse health effects other than carcinogenicity.
Risk scientists rely on a number of reputable sources to gather, compile, and analyze hazard identification information to be able to perform weight of evidence analysis and to conclude whether a chemical may cause a health effect in humans. Some of these sources are:
- Hazardous Substances Data Bank (HSDB) maintained by the National Library of Medicine: This scientifically peer-reviewed data bank provides human and animal toxicity data for about 5,000 chemicals and can be accessed via http://toxnet.nlm.nih.gov/cgi-bin/sis/htmlgen?HSDB
- ChemicIDplus Advanced database maintained by the National Library of Medicine: This database allows users to search the NLM ChemIDplus database of over 370,000 chemicals. Compound identifiers such as Chemical Name, CAS Registry Number, Molecular Formula, Classification Code, Locator Code, and Structure or Substructure can be entered to display toxicity data via http://chem.sis.nlm.nih.gov/chemidplus/
- National Toxicology Program (NTP) of the Department of Health and Human Services:
- Report of Carcinogens (RoC): The RoC is an informational scientific and public health document that identifies and discusses agents, substances, mixtures, or exposure circumstances that may pose a hazard to human health by virtue of their carcinogenicity. The RoC is published biennially and serves as a meaningful and useful compilation of data on: a) the carcinogenicity (ability to cause cancer), genotoxicity (ability to damage genes), and biologic mechanisms (modes of action in the body) of the listed substance in humans and/or animals; b) the potential for human exposure to these substances; and c) Federal regulations to limit exposures. The link to the most recent version of the RoC can be accessed via http://ntp.niehs.nih.gov/?objectid=BD1A20B5-F1F6-975E-7CF8CBFACF0FC7EF
- NTP Toxicity Testing Study Results and Research Areas: NTP tests chemicals for their toxicity in human and animal systems. The results of these toxicity testing studies along with current research areas can be obtained at: http://ntp.niehs.nih.gov/index.cfm?objectid=720160DB-BDB7-CEBA-F7CC2DE0A230C920
- National Institute of Occupational and Safety Health (NIOSH) Hazard Identification Databases: The following NIOSH website houses a multitude of databases and information for chemicals and their hazards under a single umbrella, including NIOSH’s “Pocket Guide to Chemical Hazards”: http://www.cdc.gov/niosh/database.html
- Agency for Toxic Substances and Disease Registry (ATSDR) Toxicological Profiles and Public Health Statements: ATSDR produces “toxicological profiles” for hazardous substances found at National Priorities List (NPL) Superfund sites. About 300 toxicological profiles have so far been published or are under development. The chemical-specific toxicological profiles can be accessed via http://www.atsdr.cdc.gov/toxprofiles/index.asp
- World Health Organization (WHO) International Programme of Chemical Safety (IPCS): ICPS publishes “Environmental Health Criteria” (EHC) for chemical substances, which provide critical reviews on the effects of chemicals or combinations of chemicals and physical and biological agents on human health and the environment. The IPCS site can be accessed via http://www.who.int/ipcs/assessment/en/
- Material Safety Data Sheets (MSDS): MSDS are invaluable resource to obtain compositional data for products and mixtures.
Toxicity (Dose-Response Assessment)
Dose-response assessment takes the toxicity data gathered in the hazard identification step from animal studies and exposed human population studies and describes the quantitative relationship between the amount of exposure to a chemical (or dose) and the extent of toxic injury or disease (or response). Generally, as the dose of a chemical increases, the toxic response increases either in the severity of the injury or in the incidence of response in the affected population (EPA, 1989; EPA, 1993). In toxicity-assessment step, the relationship between the magnitude of the administered, applied, or absorbed dose and the probability of occurrence and magnitude of health effect(s) (e.g. tumor incidence in the case of cancer) is determined.
Dose-response assessment for carcinogens and non-carcinogens differ in toxicity values use and how these toxicity values are derived. In general, toxicity values provide a measure of toxic potency of the chemical in question. These toxicity values are:
- Reference Dose (RfD) for oral/dermal pathways or Reference Concentration (RfC) for inhalation pathway – Noncarcinogens: A chronic RfD is defined as an estimate (with uncertainty spanning perhaps an order of magnitude or greater) of a daily exposure level for the human population, including sensitive subpopulations, that is likely to be without an appreciable risk of deleterious effects during a lifetime. It has the unit of mg of pollutant per kg of body weight per day (mg/kg-day). Chronic RfDs are specifically developed to be protective for long-term exposure to a chemical, usually, for exposure periods between seven years (approximately 10 percent of a human lifetime) and a lifetime. After selection of a critical health effect study and a critical health effect through review of toxicity literature in the hazard identification step, the RfD is derived by dividing the NOAEL (or LOAEL) for the critical toxic effect by uncertainty factors (UFs) and a modifying factor (MF). The uncertainty factors generally consist of multiples of 10, with each factor representing a specific area of uncertainty inherent in the extrapolation from the available data (e.g. 10 for extrapolation from animals to humans; 10 for interhuman variability; 10 when LOAEL is used instead of NOAEL in deriving RfD; 10 when NOAEL is obtained from a subchronic study rather than a chronic study). A modifying factor ranging from >0 to 10 is included to account for additional uncertainties in the critical study and in the entire data based on a qualitative professional assessment. The default value for the MF is 1.The NOAEL is selected based on the assumption that if the critical toxic effect is prevented, then all toxic effects are prevented (EPA, 1989). The derivation of toxicity value, RfD/RfC, for noncarcinogens assumes that they are threshold chemicals, meaning there is a threshold below which no adverse effects are observed in test species. This dose level (i.e. NOAEL) in animals is simply adjusted by a number of factors (UFs and MF) to determine the safe dose level in humans (i.e. RfD) as shown by the following equation:
- Cancer Slope Factor (CSF) for oral/dermal pathway or Unit Risk Factor (URF) for inhalation pathway – Carcinogens: Unlike the noncarcinogens, carcinogens assumed to be non-threshold chemicals based on the Environmental Protection Agency (EPA) assumption that a small number of molecular events can evoke changes in a single cell that can lead to uncontrolled cellular proliferation and eventually to cancer. In deriving slope factors, firstly, an appropriate dose-response data set is selected. In this exercise, whenever available, human data of high quality are preferable to animal data. However, if only animal data are available, dose-response data from species that responds most similarly to humans with respect to metabolism, physiology, and pharmacokinetics is preferred. When no clear choice is possible, the most sensitive species is chosen. Secondly, a model to the available data set is applied and extrapolation from the relatively high doses administered to test species in animal bioassay (or the exposures recorded in epidemiologic studies) to the lower environmental exposure levels expected for humans is performed using the model. Although various models have been developed for this purpose (e.g. probit, logit, Weibull), the linearized multistage model has commonly been used by the EPA. After the data are fit to the appropriate model, the upper 95th percent confidence limit of the slope of the resulting dose-response curve is calculated, which is known as the Cancer Slope Factor (CSF). It represents an upper 95th percent confidence limit on the probability of a response per unit intake of a chemical over a lifetime (i.e. dose). Thus, its units are (mg/kg-day) -1. This indicates that there is only a five percent chance that the probability of a response could be greater than the estimated value of CSF. Because the dose-response curve generally is linear only in the low-dose region, the slope factor estimate only holds true for low doses. Toxicity values for carcinogenic effects also can be expressed in terms of risk per unit concentration of the chemical, which are called unit risk factors (URFs). They are calculated by dividing the CSF by adult body weight (70 kg) and multiplying by the adult inhalation rate (20 m3/day), for risk associated with unit concentration in air (EPA, 1989).
A number of regulatory agencies responsible for environmental and public health protection have devoted resources in developing and documenting toxicity values for noncarcinogens (RfDs/RfCs) and carcinogens (CSFs/URFs). The following hierarchy of sources is recommended by the EPA in evaluating chemical toxicity for Superfund sites (EPA, 2003):
- Integrated Risk Information System (IRIS) and cited references, which is the prime source for the chemical-specific toxicity value information and can be accessed via: http://cfpub.epa.gov/ncea/iris/index.cfm
- The Provisional Peer Reviewed Toxicity Values (PPRTV) and cited references developed for the EPA Office of Solid Waste and Emergency Response (OSWER) Office of Superfund Remediation and Technology Innovation (OSRTI) programs (not publicly available).
- Other toxicity values, which includes the following sources of toxicity values that are commonly consulted by the EPA Superfund Program when a relevant toxicity value is not available from either IRIS or the PPRTV database:
- California Environmental Protection Agency (Cal EPA) Toxicity Criteria Database, available at: http://www.oehha.ca.gov/risk/chemicalDB/index.asp;
- The Agency for Toxic Substances and Disease Registry (ATSDR) Minimal Risk Levels (MRLs, addressing noncancer effects only). MRL is an estimate of the daily human exposure to a hazardous substance that is likely to be without appreciable risk of adverse noncancer health effects over a specified duration of exposure. These substance-specific estimates, which are intended to serve as screening levels, are used by ATSDR health assessors and other responders to identify contaminants and potential health effects that may be of concern at hazardous waste sites. To date, 137 inhalation MRLs, 226 oral MRLs and 8 external radiation MRLs have been derived and can be found at: http://www.atsdr.cdc.gov/mrls/index.html;
- The EPA Superfund Health Effects Assessment Summary Tables (HEAST) database and cited references; and
- Additional sources of toxicity values.
There are a number of other valuable sources for toxicity values (RfDs/RfCs for non-carcinogens and URFs/CSFs for carcinogens), which can be compiled via the following sources:
- EPA Region 9 tabulated “Preliminary Remediation Goals (PRGs)” or “Regional Screening Levels (RSL)” for Chemical Contaminants at Superfund Sites,” which also lists toxicity values (oral/inhalation RfD and oral/inhalation CSF) used in the medium-specific PRG/RSL calculation for each chemical. This table can be accessed via http://www.epa.gov/region09/waste/sfund/prg/index.html
- The Hot Spot Guidelines published by California EPA for Air Toxics Program includes technical background documentation for toxicity criteria/values for chemicals (i.e. Cancer Potency Factors (CPFs), which is equivalent to EPA’s CSFs and Chronic Recommended Exposure Limits (RELs), which are similar to USEPA’s RfCs). The most recent version of REL Table is located at: http://www.oehha.org/air/allrels.html. The Technical Support Document for CPFs that contains cancer unit risks and potency factors for 121 of the 201 carcinogenic substances or groups of substances can be accessed via http://www.oehha.ca.gov/air/cancer_guide/TSD2.html.
- The Department of Energy’s Oak Ridge National Laboratory (ORNL) maintains Risk Assessment Information System (RAIS) website, which contains useful information for risk assessment, including chemical-specific toxicity values. The RAIS information can be accessed via http://rais.ornl.gov/.
- Toxicology Excellence in Risk Assessment (TERA), a non-profit organization, manages and distributes a free Internet database of human health risk values and cancer classifications for over 600 chemicals of environmental concern from multiple organizations worldwide. This database, Integrated Toxicity Estimates for Risk (ITER), can be accessed via: http://www.tera.org/iter/ or via NLM’s TOXNET database at: http://toxnet.nlm.nih.gov.
The dermal RfDs and CSFs can be derived from oral RfDs and CSFs, adjusted for chemical-specific gastrointestinal absorption efficiency, based on the recommended methodology in EPA’s Guidance for Dermal Risk Assessment (EPA, 2004a).
Exposure Assessment
In the third step of risk assessment, the magnitude of exposure is determined by measuring or estimating the amount of an agent to which humans are exposed (i.e. exposure concentration) and the magnitude of dose (or intake) is estimated by taking the magnitude, frequency, duration, and route of exposure into account. Exposure assessments may consider past, present, and future exposures.
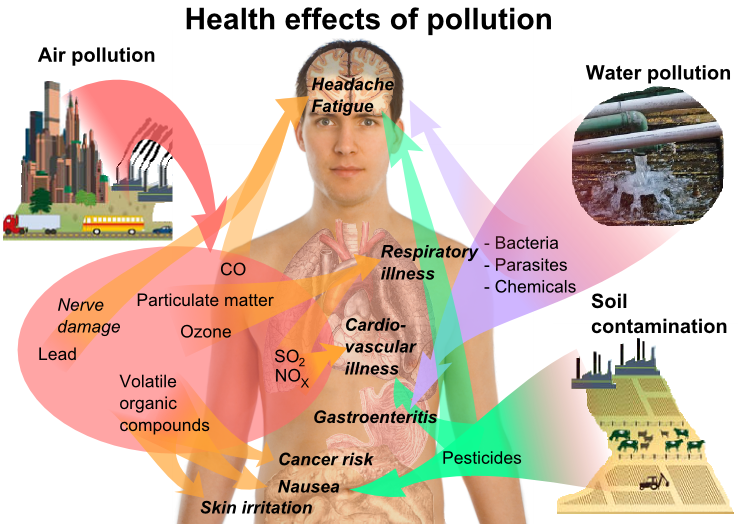
Human Health Effects of Environmental Pollution from Pollution Source to Receptor Figure shows the human health effects of environmental pollution from pollution source to receptor. Source: Mikael Häggström via Wikimedia Commons
While estimates of past or current exposure concentration/dose can be based on measurements or models of existing conditions, estimates of future exposure concentration/dose can be based on models of future conditions. In the case of inhalation exposures, personal or area monitoring to sample for contaminants in the air can be employed. The sampling data can be augmented with modeling efforts using default and/or site-specific input parameters. The model application can begin with simple screening level dispersion models and/or can utilize higher-level 2-D or 3-D models depending on the complexity of the environmental pollution problem in hand.
In any exposure assessment, the risk scientists ask a number of questions to hypothesize the exposure scenario pertaining to environmental pollution affecting the population or a sub-group. Some of these are:
- What is the source of pollution at the site? (e.g. underground storage tank leak, emissions from an industrial plant, surface water run-off from agricultural fields)
- Which environmental compartments are likely to be contaminated? (i.e. air, water, sediment, soil, plants, animals, fish)
- What are the chemicals of concern (COC) originating from the pollution source?
- What are the fate and transport properties of these chemicals that may inform the aging of the pollution in the environment over time and resultant chemical signature in each environmental medium?
- Who is exposed? (e.g. children, elderly, asthmatics, general population, workers)
- How many people are exposed?
- Where are people exposed? (e.g. home, workplace, outside environment, retirement communities, schools)
- How are people exposed? (i.e. exposure pathway – inhalation, dermal contact or ingestion)
- How often are people exposed? (i.e. exposure frequency)
- How long are people exposed? (i.e. exposure duration)
Answers to these questions frame the problem at hand. In the next step, a number of exposure parameters are integrated into an estimate of daily dose received by an exposed individual via each exposure route (ingestion, dermal contact or skin absorption, and inhalation). The magnitude of human exposures, in general, is dependent on COC concentration in soil, exposure parameters describing human physiology (e.g. soil ingestion rate, body weight), and population-specific parameters describing exposure behavior (exposure frequency, duration). When evaluating subchronic or chronic exposures to noncarcinogenic chemicals, dose is averaged over the period of exposure, termed “Average Daily Dose” (ADD). However, for carcinogens, dose is averaged over an entire lifetime (i.e. 70 years), thus referred to as “Lifetime Average Daily Dose” (LADD). Both ADD and LADD represent normalized exposure rate in the units of mg of chemical per kg body weight per day (mg/kg-day). The ADD for noncarcinogenic COCs and LADD for carcinogenic COCs are estimated for four most commonly studied exposure pathways in EPA risk assessments, particularly for hazardous waste sites, as shown below (Erdal, 2007):
Soil Ingestion:
[latex]L\left(\text{ADD}{\right)}_{o}=\frac{{C}_{s}{\text{xIR}}_{o}\text{xEFxEDxCF}}{\text{BWxAT}}[/latex]
Dermal Contact:
[latex]L\left(\text{ADD}{\right)}_{d}=\frac{{C}_{s}\text{xSAxAFxABSxEVxEFxEDxCF}}{\text{BWxAT}}[/latex]
Inhalation of Particulates:
[latex]L\left(\text{ADD}{\right)}_{\text{ip}}=\frac{{C}_{s}{\text{xIR}}_{i}\text{xEFxEDx}\left(\frac{1}{\text{PEF}}\right)}{\text{BWxAT}}[/latex]
Inhalation of Volatiles:
[latex]L\left(\text{ADD}{\right)}_{\text{iv}}=\frac{{C}_{s}{\text{xIR}}_{i}\text{xEFxEDx}\left(\frac{1}{\text{VF}}\right)}{\text{BWxAT}}[/latex]
Where:
Cs: Exposure Concentration (i.e., 95th Upper Confidence Limit on the Mean) of COC in soil (mg/kg) – (chemical-specific; can be estimated using EPA 2004b)
IRo: Ingestion rate of soil (mg/d)
IRi: Inhalation rate (m3/d)
SA: Skin surface area (cm2)
AF: Soil-to-skin adherence factor (mg/cm2)
ABS: Dermal absorption fraction (unitless – chemical-specific)
EV: Event frequency (events/d)
EF: Exposure frequency (d/y)
ED: Exposure duration (y)
PEF: Particulate emission factor (m3/kg) – 1.36 x 109 m3/kg per (EPA 2002a)
VF: Soil-to-air volatilization factor (m3/kg – chemical-specific)
BW: Body weight (kg)
AT: Averaging time (days) – (ED*365 d/y for noncarcinogens; 70 y*365 d/y for carcinogens)
CF: Conversion factor – 10-6 kg/mg
In deterministic risk assessment, ADD and LADD estimates are performed for a reasonable maximum exposure scenario (RME) and a central tendency exposure scenario (CTE), resulting in a range. EPA’s reliance on the concept of RME for estimating risks is based on a conservative but plausible exposure scenario (which is defined to be the 90th to 95th percentile exposure, signifying that fewer than five percent to 10 percent of the population would be expected to experience higher risk levels), and has been scientifically challenged over the years. For example, Burmaster and Harris (1993) showed that the use of EPA recommended default exposure parameter values resulted in exposure and risk estimates well in excess of the 99th percentile due to multiplication of three upper-bound values (i.e. 95th percentiles) for IR, EF, and ED. The authors argued that this leads to hazardous waste site cleanup decisions based on health risks that virtually no one in the surrounding population would be expected to experience. They advised the EPA to endorse and promote the use of probabilistic methods (e.g. Monte-Carlo simulations) as a way to supplement or replace current risk assessment methods, in order to overcome the problem of “compounded conservatism” and enable calculation of risks using a more statistically defensible estimate of the RME. In probabilistic risk assessment, the input parameters are characterized by their unique probability distribution. The EPA’s Exposure Factors Program provides information on development of exposure parameter distributions in support of probabilistic distributions and can be accessed via: http://cfpub.epa.gov/ncea/cfm/recordisplay.cfm?deid=20563.
The values of the exposure parameters corresponding to RME or CTE scenarios are often compiled from EPA’s Exposure Factors Handbook (EFH):
- General Exposure Factors Handbook (EPA, 1997) provides exposure assessors with data needed on standard factors to calculate human exposure to toxic chemicals as part of risk assessments. These factors include: drinking water consumption, soil ingestion, inhalation rates, dermal factors including skin area and soil adherence factors, consumption of fruits and vegetables, fish, meats, dairy products, homegrown foods, breast milk intake, human activity factors, consumer product use, and residential characteristics. Recommended values are for the general population and also for various segments of the population who may have characteristics different from the general population. The most recent version of the EFH can be accessed via http://cfpub.epa.gov/ncea/cfm/recordisplay.cfm?deid=209866.
- The Children-Specific EFH (EPA, 2002b) provides a summary of the available and up-to-date statistical data on various factors assessing child exposures, which can be accessed via http://cfpub.epa.gov/ncea/cfm/recordisplay.cfm?deid=56747.
Risk Characterization
In the last step, a hazard quotient (HQ) as an indicator of risks associated with health effects other than cancer and excess cancer risk (ECR) as the incremental probability of an exposed person developing cancer over a lifetime, are calculated by integrating toxicity and exposure information, as shown below. If HQ > 1, there may be concern for potential adverse systemic health effects in the exposed individuals. If HQ ≤ 1, there may be no concern. It should be noted that HQs are scaling factors and they are not statistically based. The EPA’s acceptable criterion for carcinogenic risks is based on public policy as described in the National Contingency Plan (NCP) and is the exposure concentration that represent an ECR in the range of 10-4 – 10-6, i.e. 1 in 10,000 to 1 in 1,000,000 excess cancer cases (EPA, 1990).
Noncancer Risk:
[latex]\text{HazardQuotient}\left(\text{HQ}\right)=\frac{\text{ADD}}{\text{RfD}}[/latex]
Excess Cancer Risk (ECR):
[latex]\text{ECR}=L\left(\text{ADD}\right)\text{xCSF}[/latex]
To account for exposures to multiple COCs via multiple pathways, individual HQs are summed to provide an overall Hazard Index (HI). If HI >1, COCs are segregated based on their critical health end-point and separate target organ-specific HIs are calculated. Only if target organ-specific HI > 1, is there concern for potential health effects for that end-point (e.g. liver, kidney, respiratory system).
Cumulative Noncancer Risk:
[latex]\text{HazardIndex}=\text{HI}=\sum _{{\text{COC}}_{\text{NC}}=1}^{n}\left({\text{HQ}}_{o}+{\text{HQ}}_{d}+{\text{HQ}}_{i}\right)[/latex]
Cumulative Excess Cancer Risk:
[latex]\sum _{{\text{COC}}_{C}=1}^{n}\text{ECR}=\sum _{{\text{COC}}_{C}=1}^{n}\left({\text{ECR}}_{o}+{\text{ECR}}_{d}+{\text{ECR}}_{i}\right)[/latex]
Here, o, d and i subscripts express oral (ingestion), dermal contact and inhalation pathways.
As discussed above, the HQ, HI, and ECR estimates are performed for RME and CTE scenarios separately in the case of deterministic risk assessment. Although EPA published the probabilistic risk assessment guidelines in 2001 (EPA, 2001), its application has so far been limited. Proper evaluation of uncertainties, which are associated with compounded conservatism and potential underestimation of quantitative risk estimates (e.g. due to the presence of COCs without established toxicity values), is intrinsic to any risk-based scientific assessment. In general, uncertainties and limitations are associated with sampling and analysis, chemical fate and transport, exposure parameters, exposure modeling, and human dose-response or toxicity assessment (derivation of CSFs/RfDs, extrapolation from high animal doses to low human doses), and site-specific uncertainties.
Conclusion
The improvement in the scientific quality and validity of health risk estimates depends on advancements in our understanding of human exposure to, and toxic effects associated with, chemicals present in environmental and occupational settings. For example, life-cycle of and health risks associated with pharmaceuticals in the environment is poorly understood due to lack of environmental concentration and human exposure data despite extensive toxicological data on drugs. There are many other examples for which either data on exposure or toxicity or both have not yet been developed, preventing quantitative assessment of health risks and development of policies that protect the environment and public health at the same time. Therefore, it is important to continue to develop research data to refine future risk assessments for informed regulatory decision-making in environmental sustainability and to ensure that costs associated with different technological and/or engineering alternatives are scientifically justified and public health-protective. One area that, particularly, requires advancement is the assessment of health risks of chemical mixtures. Current risk assessment approaches consider one chemical at a time. However, chemicals are present in mixtures in the environment. Furthermore, physical, chemical and biological transformations in the environment and interactions among chemicals in the environment may change the toxic potential of the mixture over time. Thus, risk assessment is an evolving scientific discipline that has many uncertainties in all of the four steps. These uncertainties should be thoroughly documented and discussed and the risk assessment results should be interpreted within the context of these uncertainties.
Review Questions
What are the human health hazards of vinyl chloride?
What are the human toxicity values (RfD, CSF) of vinyl chloride and how are these values estimated?
How do you calculate the dose received by children and adults via ingestion of vinyl chloride-contaminated drinking water under the RME scenario? Please document and explain your assumptions along with your references for the exposure parameters for each receptor of concern
How do you calculate RME cancer and noncancer risks to children and adults for the above exposure scenario?
What does excess cancer risk of three cases out of ten thousand exposed (3×10-4) signify?
If drinking water were also contaminated with benzene, how would you estimate cumulative cancer and noncancer risks associated with exposure to drinking water contaminated with vinyl chloride and benzene for children and adults under the RME scenario?
References
Burmaster, D.E. & Harris, R.H. (1993). The magnitude of compounding conservatisms in Superfund risk assessments. Risk Analysis, 13, 131-34.
EPA (U.S. Environmental Protection Agency). (1989). Risk assessment guidance for Superfund, volume I: Human health evaluation manual (Part A) (Interim Final) (EPA/540/1-89002). Office of Emergency and Remedial Response, Washington, DC
EPA (U.S. Environmental Protection Agency). (1990, March 8). National contingency plan. Federal Register, 55, 8848. Washington, DC.
EPA (U.S. Environmental Protection Agency). (1993, September). SI 400: Introduction to risk assessment and risk management for hazardous air pollutants. Air Pollution Training Institute. Environmental Research Center, Research Triangle Park, NC.
EPA (U.S. Environmental Protection Agency). (1997, August). Exposure factors handbook, volume I – General factors (EPA/600/P-95/002Fa). National Center for Environmental Assessment, Office of Research and Development, Washington, DC.
EPA (U.S. Environmental Protection Agency). (2001, December). Risk assessment guidance for Superfund, volume III – Part A: Process for conducting probabilistic risk assessment (EPA540-R-02-002). Office of Emergency and Remedial Response, Washington, DC.
EPA (U.S. Environmental Protection Agency). (2002a, December). Supplemental guidance for developing soil screening levels for Superfund sites (OSWER 9355.4-24). Office of Emergency and Remedial Response, Washington, DC.
EPA (U.S. Environmental Protection Agency). (2002b, September). Child-specific exposure factors handbook (EPA-600-P-00-002B). Interim Report. National Center for Environmental Assessment, Office of Research and Development, Washington, DC.
EPA (U.S. Environmental Protection Agency). (2003, December 5). Human health toxicity values in Superfund risk assessments (OSWER Directive 9285.7-53). Memorandum from Michael B. Cook, Director of Office of Superfund Remediation and Technology Innovation to Superfund National Policy Managers, Regions 1 – 10. Office of Emergency and Remedial Response, Washington, DC.
EPA (U.S. Environmental Protection Agency). (2004a, July). Risk assessment guidance for Superfund, volume I: Human health evaluation manual (Part E, supplemental guidance for dermal risk assessment) (EPA/540/R/99/005). Final. Office of Superfund Remediation and Technology Innovation, Washington, DC.
EPA (U.S. Environmental Protection Agency). (2004b, April). ProUCL Version 3.00.02 user’s guide and software (EPA/600/R04/079). Prepared by Anita Singh and Robert Maichle with Lockheed Martin Environmental Services and Ashok K. Singh of University of Nevada for the U.S. Environmental Protection Agency.
Erdal, S. (2007). Case study: Multi-pathway risk assessment for adults and children living near a hazardous waste site. In M.G. Robson & W.A. Toscano (Eds.), Environmental Health Risk Assessment for Public Health (pp. 523-530). Association of Schools of Public Health.
Lilienfeld A.M. & Lilienfeld, D.E. (1980). The French influence on the development of epidemiology. Henry E Sigerist Suppl Bull Hist Med, 4, 28-42.
NAS (National Academy of Sciences). (1983). Risk assessment in the federal government: Managing the process. National Academy Press, Washington, DC.
Glossary
- bioassay
- An assay for determining the potency (or concentration) of a substance that causes a biological change in experimental animals.
- carcinogenicity
- Defines the ability or tendency to produce cancer.
- chronic reference dose (RfD)
- An estimate (with uncertainty spanning perhaps an order of magnitude) of a daily oral exposure for a chronic duration (up to a lifetime) to the human population (including sensitive subgroups) that is likely to be without an appreciable risk of deleterious effects during a lifetime. It can be derived from a NOAEL, LOAEL, or benchmark dose, with uncertainty factors generally applied to reflect limitations of the data used. Generally used in EPA’s noncancer health assessments.
- deterministic risk assessment
- Risk evaluation involving the calculation and expression of risks as single numerical values or “single point” estimates of risk, with uncertainty and variability discussed qualitatively.
- epidemiology
- The study of the distribution and determinants of health-related states or events in specified populations.
- monte-carlo method
- A repeated random sampling from the distribution of values for each of the parameters in a generic (exposure or dose) equation to derive an estimate of the distribution of (doses or risks in) the population.
- probabilistic risk assessment
- Risk evaluation involving the calculation and expression of risks using multiple risk descriptors to provide the likelihood of various risk levels. Probabilistic risk results approximate a full range of possible outcomes and the likelihood of each, which often is presented as a frequency distribution graph, thus allowing uncertainty or variability to be expressed quantitatively.
Candela Citations
- Sustainability: A Comprehensive Foundation. Authored by: Tom Theis and Jonathan Tomkin, Editors.. Provided by: OpenStax CNX. Located at: http://cnx.org/contents/1741effd-9cda-4b2b-a91e-003e6f587263@44.1. License: CC BY: Attribution. License Terms: Download for free at http://cnx.org/contents/1741effd-9cda-4b2b-a91e-003e6f587263@44.1