Discuss the role environment plays on phenotypes
In recent years, scientists have begun to research how our environment can impact our phenotypes. In this outcome, we’ll learn about a few different ways our environment can impact us.
Learning Objectives
- Explain how epistasis impacts trait expression
- Describe polygenic inheritance and how to recognize it
- Describe continuous variation and how to recognize it
- Identify gene-environment interaction and how this impacts trait expression
- Explain pleiotropy and its impact on traits in a population
Epistasis
Mendel’s studies in pea plants implied that the sum of an individual’s phenotype was controlled by genes (or as he called them, unit factors), such that every characteristic was distinctly and completely controlled by a single gene. In fact, single observable characteristics are almost always under the influence of multiple genes (each with two or more alleles) acting in unison. For example, at least eight genes contribute to eye color in humans.
In some cases, several genes can contribute to aspects of a common phenotype without their gene products ever directly interacting. In the case of organ development, for instance, genes may be expressed sequentially, with each gene adding to the complexity and specificity of the organ. Genes may function in complementary or synergistic fashions, such that two or more genes need to be expressed simultaneously to affect a phenotype. Genes may also oppose each other, with one gene modifying the expression of another.
In epistasis, the interaction between genes is antagonistic, such that one gene masks or interferes with the expression of another. “Epistasis” is a word composed of Greek roots that mean “standing upon.” The alleles that are being masked or silenced are said to be hypostatic to the epistatic alleles that are doing the masking. Often the biochemical basis of epistasis is a gene pathway in which the expression of one gene is dependent on the function of a gene that precedes or follows it in the pathway.
An example of epistasis is pigmentation in mice. The wild-type coat color, agouti (AA), is dominant to solid-colored fur (aa). However, a separate gene (C) is necessary for pigment production. A mouse with a recessive c allele at this locus is unable to produce pigment and is albino regardless of the allele present at locus A (Figure 1). Therefore, the genotypes AAcc, Aacc, and aacc all produce the same albino phenotype. A cross between heterozygotes for both genes (AaCc x AaCc) would generate offspring with a phenotypic ratio of 9 agouti:3 solid color:4 albino (Figure 1). In this case, the C gene is epistatic to the A gene.
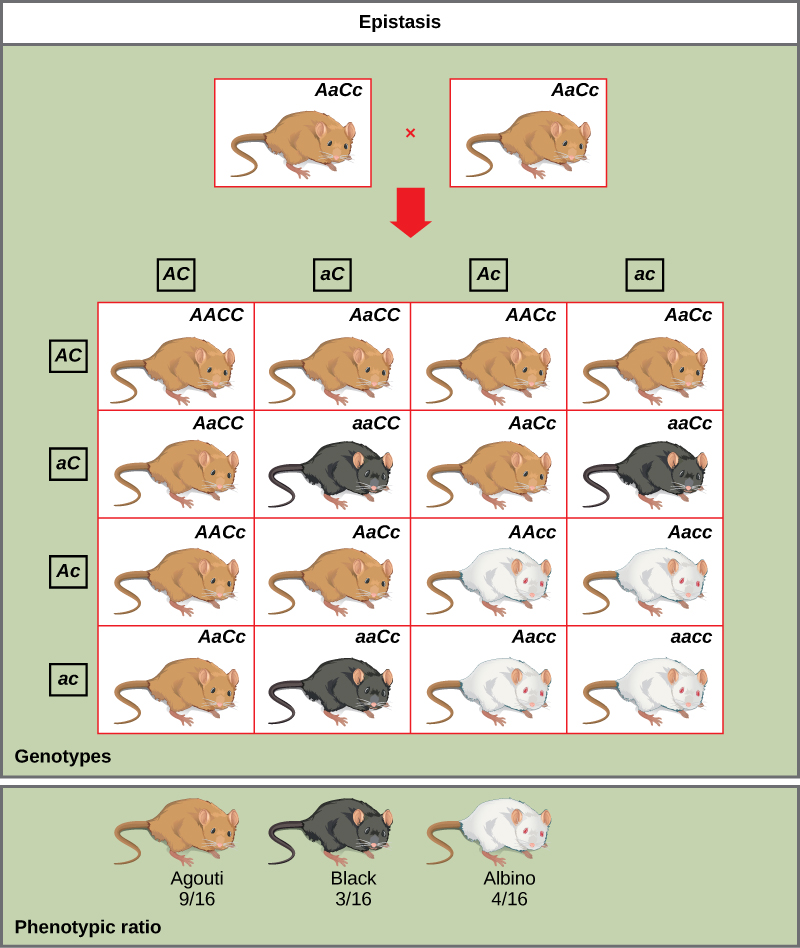
Figure 1. In mice, the mottled agouti coat color (A) is dominant to a solid coloration, such as black or gray. A gene at a separate locus (C) is responsible for pigment production. The recessive c allele does not produce pigment, and a mouse with the homozygous recessive cc enotype is albino regardless of the allele present at the A locus. Thus, the C gene is epistatic to the A gene.
Epistasis can also occur when a dominant allele masks expression at a separate gene. Fruit color in summer squash is expressed in this way. Homozygous recessive expression of the W gene (ww) coupled with homozygous dominant or heterozygous expression of the Y gene (YY or Yy) generates yellow fruit, and the wwyy genotype produces green fruit. However, if a dominant copy of the W gene is present in the homozygous or heterozygous form, the summer squash will produce white fruit regardless of the Y alleles. A cross between white heterozygotes for both genes (WwYy × WwYy) would produce offspring with a phenotypic ratio of 12 white:3 yellow:1 green.
Finally, epistasis can be reciprocal such that either gene, when present in the dominant (or recessive) form, expresses the same phenotype. In the shepherd’s purse plant (Capsella bursa-pastoris), the characteristic of seed shape is controlled by two genes in a dominant epistatic relationship. When the genes A and B are both homozygous recessive (aabb), the seeds are ovoid. If the dominant allele for either of these genes is present, the result is triangular seeds. That is, every possible genotype other than aabb results in triangular seeds, and a cross between heterozygotes for both genes (AaBb x AaBb) would yield offspring with a phenotypic ratio of 15 triangular:1 ovoid.
As you work through genetics problems, keep in mind that any single characteristic that results in a phenotypic ratio that totals 16 is typical of a two-gene interaction. Recall the phenotypic inheritance pattern for Mendel’s dihybrid cross, which considered two non-interacting genes—9:3:3:1. Similarly, we would expect interacting gene pairs to also exhibit ratios expressed as 16 parts. Note that we are assuming the interacting genes are not linked; they are still assorting independently into gametes.
Whether or not they are sorting independently, genes may interact at the level of gene products such that the expression of an allele for one gene masks or modifies the expression of an allele for a different gene. This is called epistasis.
Polygenic Inheritance and Environmental Effects
How is Height Inherited?
Many heritable human characteristics don’t seem to follow Mendelian rules in their inheritance patterns. For example, consider human height. Unlike a simple Mendelian characteristic, human height displays:
- Continuous variation. Unlike Mendel’s pea plants, humans don’t come in two clear-cut “tall” and “short” varieties. In fact, they don’t even come in four heights, or eight, or sixteen. Instead, it’s possible to get humans of many different heights, and height can vary in increments of inches or fractions of inches. As an example, consider the bell curve-shaped graph in Figure 2, which shows the heights of a group of male high school seniors.
- A complex inheritance pattern. If you’ve paid attention to the heights of your friends and family, you may have noticed that many different patterns of inheritance are possible. Tall parents can have a short child, short parents can have a tall child, and two parents of different heights may or may not have a child of intermediate height. In addition, siblings with the same two parents may have a range of heights, ones that don’t fall into clear, distinct categories. Simple models involving one or two genes can’t accurately predict all of these inheritance patterns.
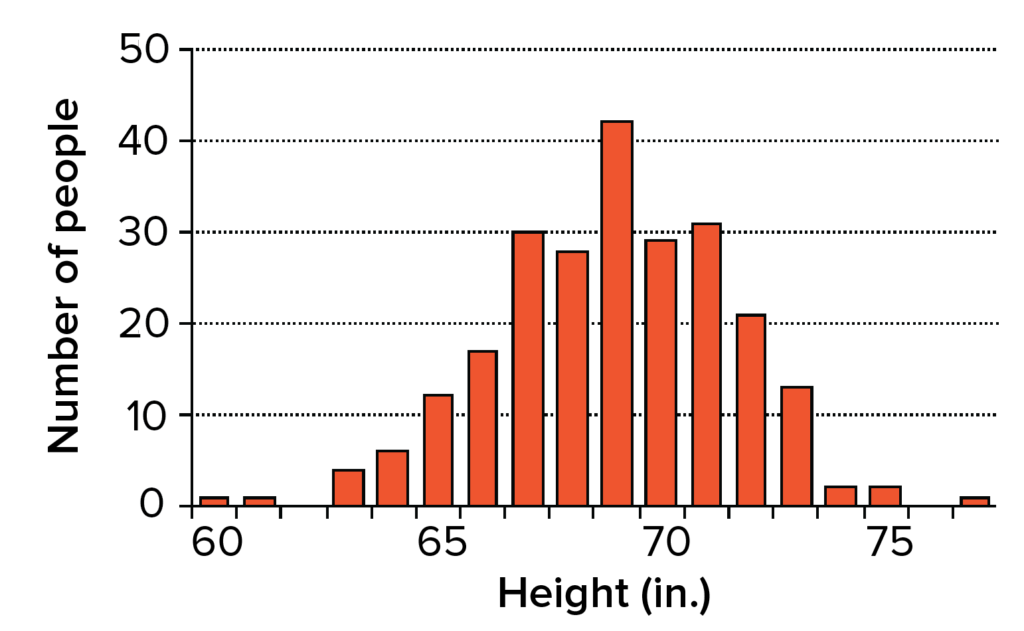
Figure 2. Heights of male high school seniors. Image modified from “Continuous variation: Quantitative traits,” by J. W. Kimball (CC BY 3.0).
How, then, is height inherited? Height and other similar features are controlled not just by one gene, but rather, by multiple (often many) genes that each make a small contribution to the overall outcome. This inheritance pattern is called polygenic inheritance (poly– = many). For instance, a recent study found over 400 genes linked to variation in height[1]. When there are large numbers of genes involved, it becomes hard to distinguish the effect of each individual gene, and even harder to see that gene variants (alleles) are inherited according to Mendelian rules. In a further complication, height doesn’t just depend on genetics: it also depends a lot on environmental factors, such as a child’s overall health and the type of nutrition he or she receives while growing up.
In this article, we’ll look in more detail at how complex human traits such as height are inherited, as well as how factors like genetic background and environment can influence the phenotype (observable features) produced by a particular genotype (set of gene variants, or alleles).
Polygenic Inheritance
Some human characteristics, such as height, eye color, and hair color, don’t come in just a few distinct forms. Instead, they vary in small gradations, forming a spectrum or continuum of possible phenotypes. Features like these are called quantitative characters, and they’re typically controlled by multiple genes (often, many genes), each of which contributes to the overall phenotype. For example, although there are two major eye color genes, there are at least 14 additional genes that play roles in determining a person’s exact eye color[2].
Looking at a real example of a human polygenic trait would get complicated, largely because we’d have to keep track of tens, or even hundreds, of different allele pairs. However, we can use an example involving the color of wheat kernels to see how Mendelian inheritance of multiple genes (plus a little incomplete dominance of alleles) can produce a broad spectrum of phenotypes[3]. In this example, there are three genes that make reddish pigment in wheat kernels, which we’ll call A, B, and C. Each comes in two alleles, one of which makes a unit of pigment (the capital-letter allele) and one of which does not make any pigment (the lowercase allele). Thus, the aa genotype would contribute zero units of pigment, the Aa genotype would contribute one unit, and the AA genotype would contribute two—basically, a form of incomplete dominance.
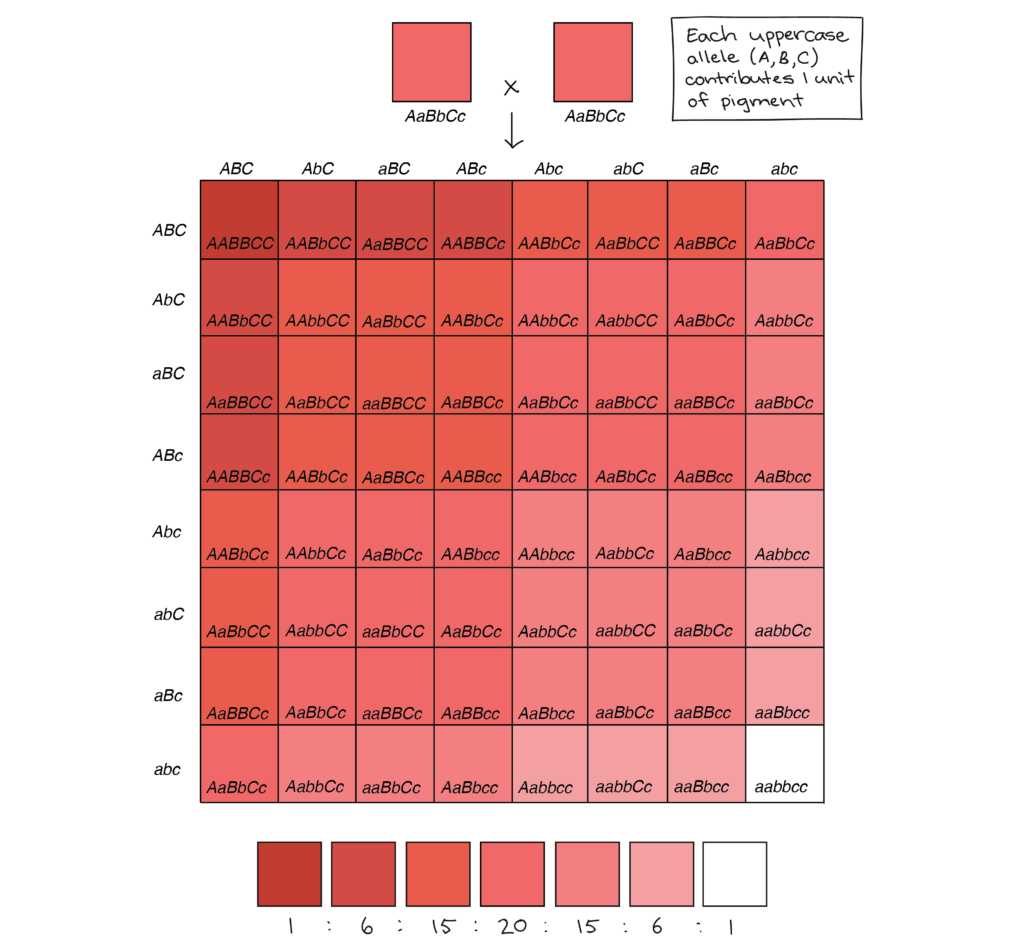
Figure 3.
Now, let’s imagine that two plants heterozygous for all three genes (AaBbCc) were crossed to one another (or, equivalently, allowed to self-fertilize). Each of the parent plants would have three units of pigment, or pinkish kernels. Their offspring, however, could display seven different categories of phenotypes, ranging from zero units of pigment (aabbcc) and pure white kernels to six units of pigment (AABBCC) and dark red kernels, with the intermediate phenotypes being most common.
This example illustrates how we can get a spectrum of slightly different phenotypes (something approaching continuous variation) with just three genes whose alleles display incomplete dominance. It’s not hard to imagine that, as we increased the number of genes involved, we’d be able to get even finer variations in color, or in another trait such as height. Real polygenic traits aren’t usually quite this clean and simple. (For instance, genes may make unequal contributions to the phenotype, alleles may or may not display incomplete dominance, and there may be non-additive interactions between genes.) However, the basic idea—that multiple genes obeying Mendelian rules can produce a spectrum of finely differing phenotypes—holds true for human traits such as skin and eye color.
PRactice Questions
We’ve learned about polygenic inheritance and continuous variation. Just what is the difference between these two types of inheritance?
Effect of the Environment
Characteristics that are influenced by environmental as well as genetic factors are called multifactorial. The idea of “nature versus nurture” — in other words, the relative influence of genetics versus environmental factors — has been and still is debated. Just looking at the genes of a given organism will not determine how that organism will develop and act. Even identical twins will show different characteristics, depending on the environment in which they live. Everyone is a product of their environment as well as their genetics.
Even when influenced by the environment, phenotypes have a normal range of expression. For instance, human height varies based on nutrition and genetics, but not many people are shorter than 4½ feet or taller than 7 feet. The range of phenotypic possibilities is called the norm of reaction. Hydrangeas, for example, may be blue, pink, or purple, but they are never naturally orange. Hydrangeas are blue in acidic soil with available aluminum, and they are pink in alkaline soil without available aluminum.
Some human characteristics have a narrow norm of reaction, such as blood type. Others have a wide norm of reaction, such as the number of blood cells in humans, which varies depending on factors that include physical fitness, presence or history of infections, and even the altitude at which a person lives.
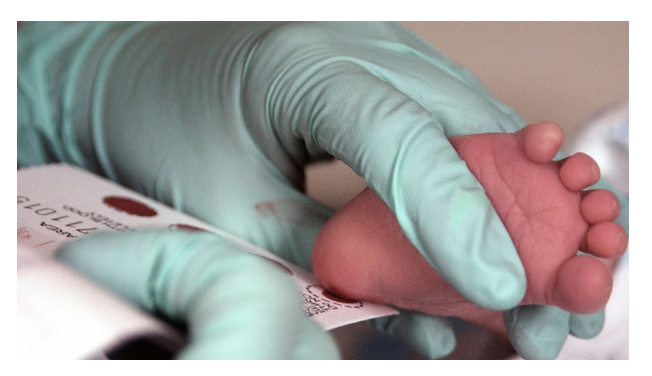
Figure 4. Taking a newborn blood sample for PKU testing. By Staff Sgt Eric T. Sheler, U.S. Air Force (Phenylketonuria testing) Public Domain
The environment also affects human genes. Serotonin, a neurotransmitter that acts inside brain cells, lowers anxiety and depression during traumatic times. Mutations in the serotonin transporter gene may cause a reduced ability to cope with stress. That does not mean that the person is always depressed, but if the environment produces stress, the person may become depressed more easily than a person with unmutated serotonin transporter genes.
You already learned about PKU, a pleiotropic disorder caused by defects in a single gene coding for an enzyme that converts the amino acid phenylalanine to tyrosine. Newborns are tested for this defect very early in life (Figure 4), so that if the results are positive, they can be given a diet limiting phenylalanine ingestion. That way, the toxic buildup is prevented and the children can develop normally. PKU is an example in which environmental factors can modify gene expression.
Practice Question
Two identical twins (female) live in different parts of the country. One is very committed to a healthy lifestyle: not smoking, exercising regularly, eating a diet rich in fresh produce, and avoiding red meats and processed foods. The other is not as careful: she smokes, is overweight, and often eats fast and processed foods. They are aware that several women in their family have had breast cancer, and decide to consult a doctor about their odds of developing the disease. Which of the following statements by the doctor sounds most correct?
- As identical twins, you are genetically the same, so your chances of developing breast cancer are identical.
- The twin with the healthy lifestyle should not be terribly concerned, while the one with the unhealthy lifestyle is at a higher risk.
- Breast cancer has a genetic component, and the twins have identical genes, so they have the same genetic risk. However, environmental factors such as smoking, obesity, and consumption of red meat have been shown to increase the risk of cancer. While both twins should monitor themselves closely, the twin who smokes and is overweight may want to consider a healthier lifestyle to decrease her risk of breast cancer.
While genes and genetic causes play a large role in health and phenotypes, the environment also plays an important role. Understanding this can enable the treatment of some disorders, such as the case with PKU in which limiting the intake of phenylalanine can prevent toxic build up of this amino acid. Often the norm of reaction is set by genetic factors but ultimately determined by environmental exposures.
Pleiotropy and Human Disorders
Based on Mendel’s experiments, you might imagine that all genes control a single characteristic, are present in two copies, and affect some harmless aspect of an organism’s appearance (such as color, height, or shape). Although those predictions are accurate in many cases, there are also some important exceptions. For instance, how can we explain observations like the following?
- The genetic disorder Marfan syndrome is caused by a mutation in one gene, yet it affects many aspects of growth and development, including height, vision, and heart function.
To understand observations like these, we need to look more deeply at what genes are. Rather than abstract “heritable factors,” genes are stretches of DNA found on chromosomes, and most of them encode (specify the sequence of) proteins that do a certain job in the cell or body. In this article, we’ll look in more detail at genes affecting multiple characteristics (pleiotropy).
Pleiotropy
When we discussed Mendel’s experiments with purple-flowered and white-flowered plants, we didn’t mention any other phenotypes associated with the two flower colors. However, Mendel noticed that the flower colors were always correlated with two other features: the color of the seed coat (covering of the seed) and the color of the axils (junctions where the leaves met the main stem)[4]. In plants with white flowers, the seed coats and axils were colorless, while in plants with purple flowers, the seed coats were brown-gray and the axils were reddish. Thus, rather than affecting just one characteristic, the flower color gene actually affected three.
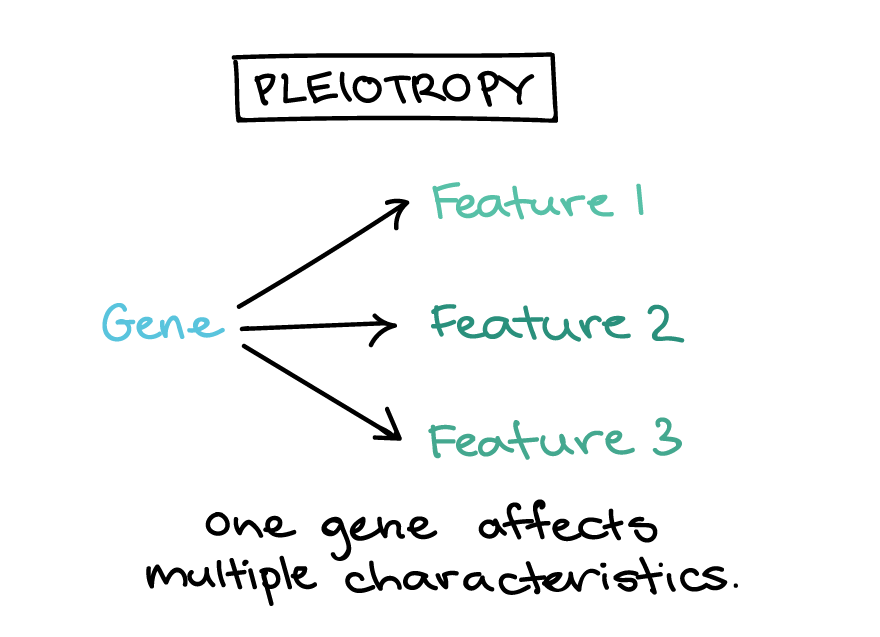
Figure 5. Based on similar diagram by Ingrid Lobo
Genes like this, which affect multiple, seemingly unrelated aspects of an organism’s phenotype, are said to be pleiotropic (pleio– = many, –tropic = effects)[5]. We now know that Mendel’s flower color gene encodes a regulator protein that activates pigment biosynthesis, and that it works in several different parts of the pea plant (flowers, seed coat, and leaf axils). Thus, the seemingly unrelated phenotypes can all be traced back to a defect in a single gene with several jobs.
Importantly, alleles of pleiotropic genes are transmitted in the same way as alleles of genes that affect single traits. Although the phenotype has multiple elements, these elements are specified as a package, and the dominant and recessive versions of the package would appear in the progeny of a monohybrid cross in a ratio of 3:1.
Pleiotropy in Human Genetic Disorders
Genes affected in human genetic disorders are often pleiotropic. For example, people with the hereditary disorder Marfan syndrome may have a constellation of seemingly unrelated symptoms[6]:
- Unusually tall height
- Thin fingers and toes
- Dislocation of the lens of the eye
- Heart problems (in which the aorta, the large blood vessel carrying blood away from the heart, bulges or ruptures).
These symptoms don’t appear directly related to one another, but as it turns out, they can all be traced back to the mutation of a single gene. This gene encodes a protein that assembles into chains, making elastic fibrils that give strength and flexibility to the body’s connective tissues[7]. Disease-causing mutations in the Marfan syndrome reduce the amount of functional protein produced, resulting in fewer fibrils. The eye and the aorta normally contain many fibrils that help maintain structure, explaining why these two organs are strongly affected in Marfan syndrome[8]. In addition, the fibrils serve as “storage shelves” for growth factors. When there are fewer of them in Marfan syndrome, the growth factors cannot be shelved and thus cause excess growth (leading to the characteristic tall, thin Marfan build)[9].
Check Your Understanding
Answer the question(s) below to see how well you understand the topics covered in the previous section. This short quiz does not count toward your grade in the class, and you can retake it an unlimited number of times.
Use this quiz to check your understanding and decide whether to (1) study the previous section further or (2) move on to the next section.
- Wood, A. R., Esko, T., Yang, J., Vedantam, S., Pers, T. H., Gustafsson, S., ... Frayling, T. M. (2014). Defining the role of common variation in the genomic and biological architecture of adult human height. Nature Genetics, 46, 1173–1186. http://dx.doi.org/10.1038/ng.3097. ↵
- White, D. and Rabago-Smith, M. (2011). Genotype-phenotype associations and human eye color. Journal of Human Genetics, 56, 5–7. http://dx.doi.org/10.1038/jhg.2010.126. ↵
- Kimball, J. W. (2011, March 8). Continuous variation: Quantitative traits. Retrieved from http://users.rcn.com/jkimball.ma.ultranet/BiologyPages/Q/QTL.html. ↵
- Lobo, I. (2008). Pleiotropy: One gene can affect multiple traits. Nature Education, 1(1), 10. Retrieved from http://www.nature.com/scitable/topicpage/pleiotropy-one-gene-can-affect-multiple-traits-569. ↵
- Ibid. ↵
- Marfan syndrome. (2012). In Genetics home reference. Retrieved from http://ghr.nlm.nih.gov/condition/marfan-syndrome. ↵
- FBN1. (2015). In Genetics home reference. Retrieved from http://ghr.nlm.nih.gov/gene/FBN1. ↵
- Marfan syndrome. (2015, November 3). Retrieved November 21, 2015 from Wikipedia: https://en.wikipedia.org/wiki/Marfan_syndrome. ↵
- FBN1. (2015). In Genetics home reference. Retrieved from http://ghr.nlm.nih.gov/gene/FBN1. ↵