Learning Outcomes
- Explain the stages of an action potential and how action potentials are propagated
A neuron can receive input from other neurons and, if this input is strong enough, send the signal to downstream neurons. Transmission of a signal between neurons is generally carried by a chemical called a neurotransmitter. Transmission of a signal within a neuron (from dendrite to axon terminal) is carried by a brief reversal of the resting membrane potential called an action potential. When neurotransmitter molecules bind to receptors located on a neuron’s dendrites, ion channels open. At excitatory synapses, this opening allows positive ions to enter the neuron and results in depolarization of the membrane—a decrease in the difference in voltage between the inside and outside of the neuron. A stimulus from a sensory cell or another neuron depolarizes the target neuron to its threshold potential (−55 mV). Na+ channels in the axon hillock open, allowing positive ions to enter the cell (Figure 1).
Once the sodium channels open, the neuron completely depolarizes to a membrane potential of about +40 mV. Action potentials are considered an “all-or nothing” event, in that, once the threshold potential is reached, the neuron always completely depolarizes. Once depolarization is complete, the cell must now “reset” its membrane voltage back to the resting potential. To accomplish this, the Na+channels close and cannot be opened. This begins the neuron’s refractory period, in which it cannot produce another action potential because its sodium channels will not open. At the same time, voltage-gated K+ channels open, allowing K+ to leave the cell. As K+ ions leave the cell, the membrane potential once again becomes negative. The diffusion of K+ out of the cell actually hyperpolarizes the cell, in that the membrane potential becomes more negative than the cell’s normal resting potential. At this point, the sodium channels will return to their resting state, meaning they are ready to open again if the membrane potential again exceeds the threshold potential. Eventually the extra K+ ions diffuse out of the cell through the potassium leakage channels, bringing the cell from its hyperpolarized state, back to its resting membrane potential.
Practice Question
The formation of an action potential can be divided into five steps, which can be seen in Figure 1.
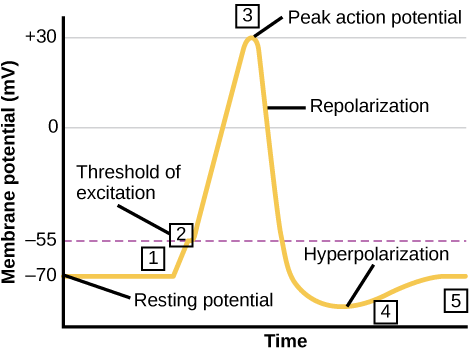
Figure 1. Action Potential
- A stimulus from a sensory cell or another neuron causes the target cell to depolarize toward the threshold potential.
- If the threshold of excitation is reached, all Na+ channels open and the membrane depolarizes.
- At the peak action potential, K+ channels open and K+ begins to leave the cell. At the same time, Na+ channels close.
- The membrane becomes hyperpolarized as K+ ions continue to leave the cell. The hyperpolarized membrane is in a refractory period and cannot fire.
- The K+ channels close and the Na+/K+ transporter restores the resting potential.
Potassium channel blockers, such as amiodarone and procainamide, which are used to treat abnormal electrical activity in the heart, called cardiac dysrhythmia, impede the movement of K+ through voltage-gated K+ channels. Which part of the action potential would you expect potassium channels to affect?
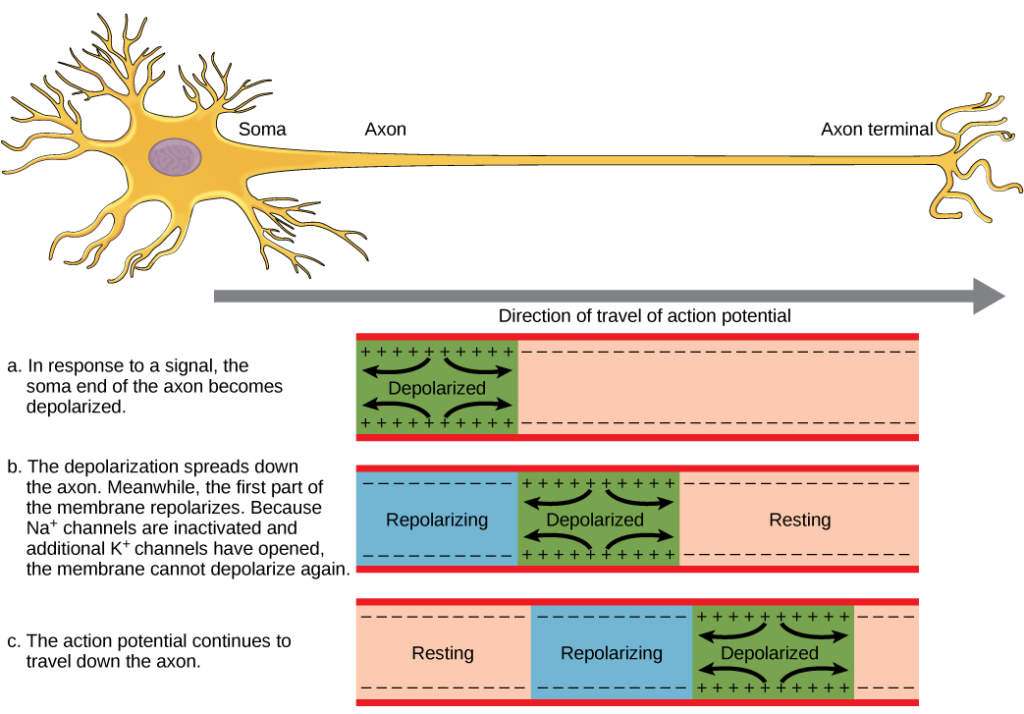
Figure 2. The action potential is conducted down the axon as the axon membrane depolarizes, then repolarizes.
Myelin and the Propagation of the Action Potential
For an action potential to communicate information to another neuron, it must travel along the axon and reach the axon terminals where it can initiate neurotransmitter release. The speed of conduction of an action potential along an axon is influenced by both the diameter of the axon and the axon’s resistance to current leak. Myelin acts as an insulator that prevents current from leaving the axon; this increases the speed of action potential conduction. In demyelinating diseases like multiple sclerosis, action potential conduction slows because current leaks from previously insulated axon areas.
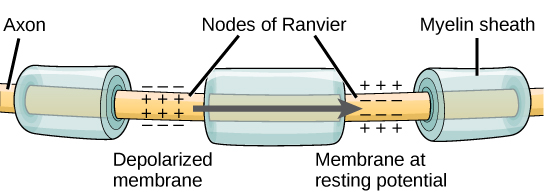
Figure 3. Nodes of Ranvier are gaps in myelin coverage along axons. Nodes contain voltage-gated K+ and Na+ channels. Action potentials travel down the axon by jumping from one node to the next.
The nodes of Ranvier, illustrated in Figure 3 are gaps in the myelin sheath along the axon. These unmyelinated spaces are about one micrometer long and contain voltage gated Na+ and K+ channels. Flow of ions through these channels, particularly the Na+ channels, regenerates the action potential over and over again along the axon. This ‘jumping’ of the action potential from one node to the next is called saltatory conduction. If nodes of Ranvier were not present along an axon, the action potential would propagate very slowly since Na+ and K+ channels would have to continuously regenerate action potentials at every point along the axon instead of at specific points. Nodes of Ranvier also save energy for the neuron since the channels only need to be present at the nodes and not along the entire axon.
Try It
Candela Citations
- Biology 2e. Provided by: OpenStax. Located at: http://cnx.org/contents/185cbf87-c72e-48f5-b51e-f14f21b5eabd@10.8. License: CC BY: Attribution. License Terms: Access for free at https://openstax.org/books/biology-2e/pages/1-introduction
- What is action potential. Provided by: Interactive Biology. Located at: https://www.youtube.com/watch?v=POSPO8QbZI4. License: All Rights Reserved