Compare and contrast stress versus strain in the Earth’s crust
This section introduces you to the concepts of stress and strain. You will learn their definitions and how they impact the Earth’s crust.
What You’ll Learn to Do
- Differentiate between the types of stress: tension, compression, shear.
- Differentiate between the types of strain: elastic, ductile, and fracture.
Stress In Earth’s Crust
Enormous slabs of lithosphere move unevenly over the planet’s spherical surface, resulting in earthquakes. This chapter deals with two types of geological activity that occur because of plate tectonics: mountain building and earthquakes. First, we will consider what can happen to rocks when they are exposed to stress.
Causes and Types of Stress
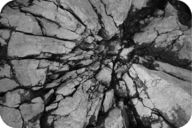
Figure 1. Stress caused these rocks to fracture.
**Stress is the force applied to an object. In geology, stress is the force per unit area that is placed on a rock. Four types of stresses act on materials.
- A deeply buried rock is pushed down by the weight of all the material above it. Since the rock cannot move, it cannot deform. This is called confining stress.
- Compression squeezes rocks together, causing rocks to fold or fracture (break) (figure 1). Compression is the most common stress at convergent plate boundaries.
- Rocks that are pulled apart are under tension. Rocks under tension lengthen or break apart. Tension is the major type of stress at divergent plate boundaries.
- When forces are parallel but moving in opposite directions, the stress is called shear (figure 2). Shear stress is the most common stress at transform plate boundaries.
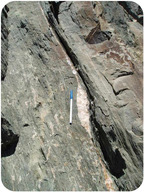
Figure 2. Shearing in rocks. The white quartz vein has been elongated by shear.
**When stress causes a material to change shape, it has undergone strain ordeformation. Deformed rocks are common in geologically active areas.
A rock’s response to stress depends on the rock type, the surrounding temperature, and pressure conditions the rock is under, the length of time the rock is under stress, and the type of stress.
Rocks have three possible responses to increasing stress (illustrated in figure 3):
- elastic deformation: the rock returns to its original shape when the stress is removed.
- plastic deformation: the rock does not return to its original shape when the stress is removed.
- fracture: the rock breaks.
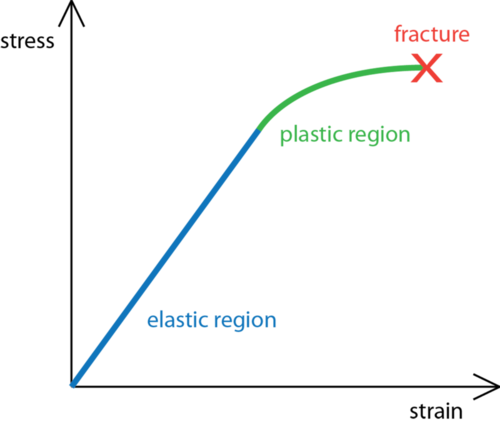
Figure 3. With increasing stress, the rock undergoes: (1) elastic deformation, (2) plastic deformation, and (3) fracture.
Under what conditions do you think a rock is more likely to fracture? Is it more likely to break deep within Earth’s crust or at the surface? What if the stress applied is sharp rather than gradual?
-
At the Earth’s surface, rocks usually break quite quickly, but deeper in the crust, where temperatures and pressures are higher, rocks are more likely to deform plastically.
-
Sudden stress, such as a hit with a hammer, is more likely to make a rock break. Stress applied over time often leads to plastic deformation.
Geologic Structures
Sedimentary rocks are important for deciphering the geologic history of a region because they follow certain rules.
- Sedimentary rocks are formed with the oldest layers on the bottom and the youngest on top.
- Sediments are deposited horizontally, so sedimentary rock layers are originally horizontal, as are some volcanic rocks, such as ash falls.
- Sedimentary rock layers that are not horizontal are deformed.
You can trace the deformation a rock has experienced by seeing how it differs from its original horizontal, oldest-on-bottom position (figure 4a). This deformation produces geologic structures such as folds, joints, and faults that are caused by stresses (figure 4b). Using the rules listed above, try to figure out the geologic history of the geologic column below.
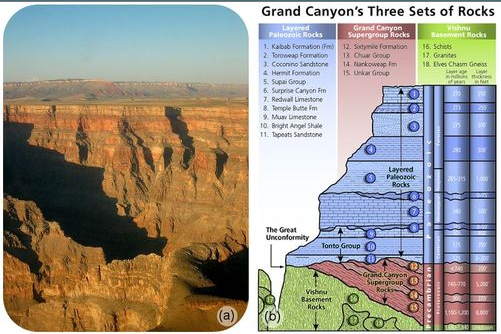
Figure 4. (a) In the Grand Canyon, the rock layers are exposed like a layer cake. Each layer is made of sediments that were deposited in a particular environment – perhaps a lake bed, shallow offshore region, or a sand dune. (b) In this geologic column of the Grand Canyon, the sedimentary rocks of the “Layered Paleozoic Rocks” column (layers 1 through 11) are still horizontal. Grand Canyon Supergroup rocks (layers 12 through 15) have been tilted. Vishnu Basement Rocks are not sedimentary (rocks 16 through 18). The oldest layers are on the bottom and youngest are on the top.
Folds
Rocks deforming plastically under compressive stresses crumple into folds (figure 5). They do not return to their original shape.
If rocks experience stress, but are in a rigid (non-plastic) state, then they may simply undergo breaking as faults and fractures.
Plastic Deformation is like silly-putty, or taffy, or cold toothpaste… if you push on it, it does not break, and instead it mushes!
Further along in this section we’ll go into more detail, but PLASTIC DEFORMATION occurs when rocks have the right combination of
- Composition
- Temperature (typically elevated temp!)
- Lithostatic pressure
- Strain rate
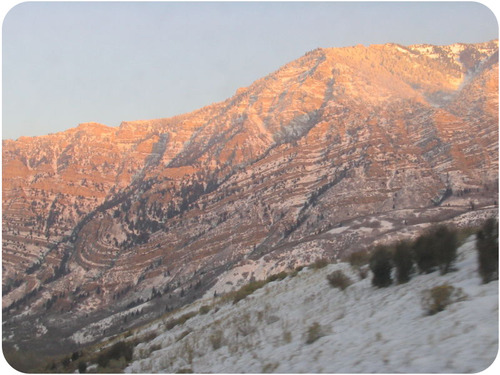
Figure 5. Snow accentuates the fold exposed in these rocks in Provo Canyon, Utah.
SPEAKING OF SILLY PUTTY— ever try this! I’ve made some big messes doing this in a classroom… so be careful.
But if you RAPIDLY apply stress (quick whack with a hammer)– silly putty does not mush; instead it breaks into lots of little pieces.
With rapid application of stress, Silly Putty is NOT a plastic (easily deformable) solid, and it breaks like a brittle solid!
Thinking Putty meets hammer (HD) – YouTube
Three types of folds are seen.
- Mononcline: A monocline is a simple bend in the rock layers so that they are no longer horizontal (see figure 6 for an example).
Figure 6. At Colorado National Monument, the rocks in a monocline plunge toward the ground.
- Anticline: An anticline is a fold that arches upward. The rocks dip away from the center of the fold (figure 7). The oldest rocks are at the center of an anticline and the youngest are draped over them.
Figure 7. (a) Schematic of an anticline. (b) An anticline exposed in a road cut in New Jersey.
When rocks arch upward to form a circular structure, that structure is called a dome. If the top of the dome is sliced off, where are the oldest rocks located?
- Syncline: A syncline is a fold that bends downward. The youngest rocks are at the center and the oldest are at the outside (figure 8).
Figure 8. (a) Schematic of a syncline. (b) This syncline is in Rainbow Basin, California.
When rocks bend downward in a circular structure, that structure is called a basin (figure 9). If the rocks are exposed at the surface, where are the oldest rocks located?
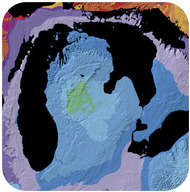
Figure 9. Basins can be enormous. This is a geologic map of the Michigan Basin, which is centered in the state of Michigan but extends into four other states and a Canadian province.
Faults
A rock under enough stress will fracture. If there is no movement on either side of a fracture, the fracture is called a joint, as shown in (figure 10).
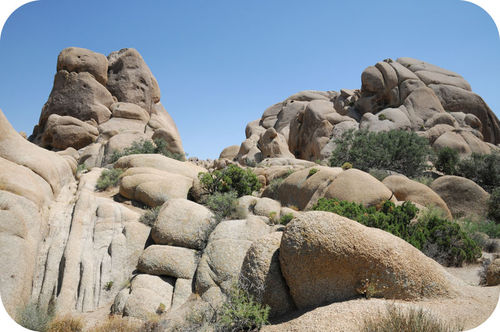
Figure 10. Granite rocks in Joshua Tree National Park showing horizontal and vertical jointing. These joints formed when the confining stress was removed from the granite.
If the blocks of rock on one or both sides of a fracture move, the fracture is called a fault (figure 11). Sudden motions along faults cause rocks to break and move suddenly. The energy released is an earthquake.
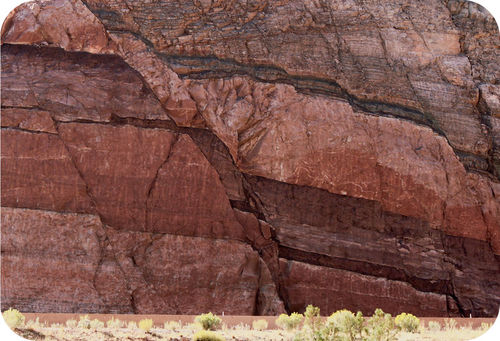
Figure 11. Faults are easy to recognize as they cut across bedded rocks.
Slip is the distance rocks move along a fault. Slip can be up or down the fault plane. Slip is relative, because there is usually no way to know whether both sides moved or only one. Faults lie at an angle to the horizontal surface of the Earth. That angle is called the fault’s dip. The dip defines which of two basic types a fault is. If the fault’s dip is inclined relative to the horizontal, the fault is a dip-slip fault (figure 12). There are two types of dip-slip faults. In normal faults, the hanging wall drops down relative to the footwall. In reverse faults, the footwall drops down relative to the hanging wall.
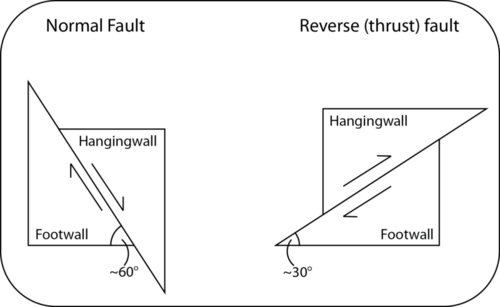
Figure 12. This diagram illustrates the two types of dip-slip faults: normal faults and reverse faults. Imagine miners extracting a resource along a fault. The hanging wall is where miners would have hung their lanterns. The footwall is where they would have walked.
Here is an animation of a normal fault.
A thrust fault is a type of reverse fault in which the fault plane angle is nearly horizontal. Rocks can slip many miles along thrust faults (Figure 13).
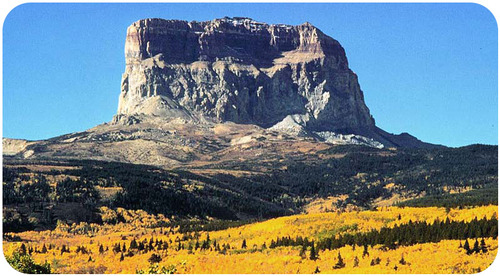
Figure 13. At Chief Mountain in Montana, the upper rocks at the Lewis Overthrust are more than 1 billion years older than the lower rocks. How could this happen?
Here is an animation of a thrust fault.
Normal faults can be huge. They are responsible for uplifting mountain ranges in regions experiencing tensional stress (figure 14).
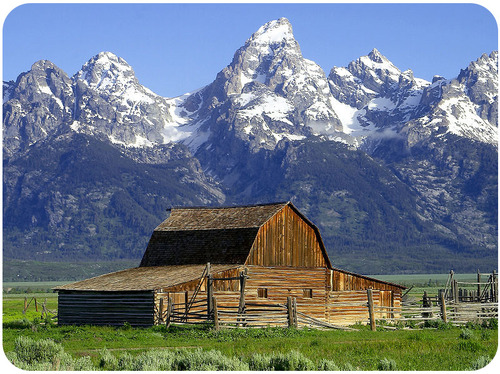
Figure 14. The Teton Range in Wyoming rose up along a normal fault.
A strike-slip fault is a dip-slip fault in which the dip of the fault plane is vertical. Strike-slip faults result from shear stresses (figure 15).
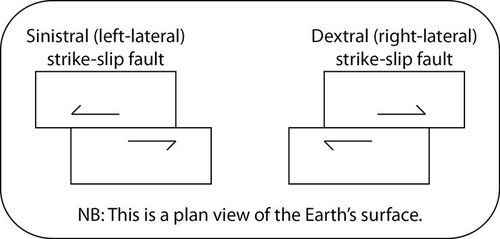
Figure 15. Imagine placing one foot on either side of a strike-slip fault. One block moves toward you. If that block moves toward your right foot, the fault is a right-lateral strike-slip fault; if that block moves toward your left foot, the fault is a left-lateral strike-slip fault.
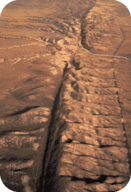
Figure 16. The San Andreas is a massive transform fault.
California’s San Andreas Fault is the world’s most famous strike-slip fault. It is a right-lateral strike slip fault (figure 16).
Here is a strike-slip fault animation.
People sometimes say that California will fall into the ocean someday, which is not true. This animation shows movement on the San Andreas into the future.
Stress and Mountain Building
Two converging continental plates smash upwards to create mountain ranges (figure 17). Stresses from this uplift cause folds, reverse faults, and thrust faults, which allow the crust to rise upwards.
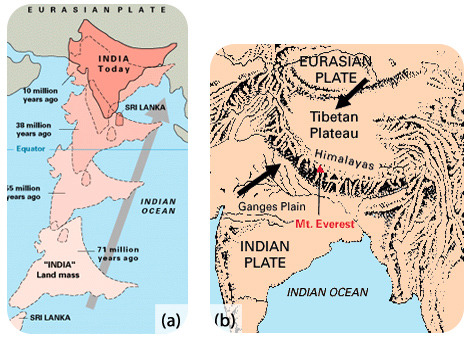
Figure 17. (a) The world’s highest mountain range, the Himalayas, is growing from the collision between the Indian and the Eurasian plates. (b) The crumpling of the Indian and Eurasian plates of continental crust creates the Himalayas.
Subduction of oceanic lithosphere at convergent plate boundaries also builds mountain ranges (figure 18).
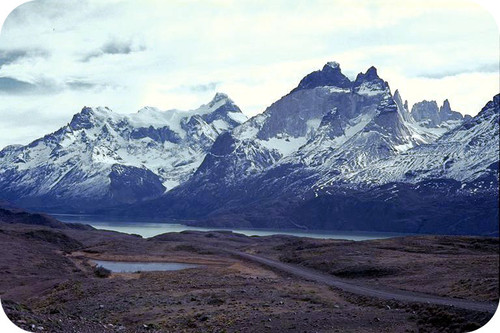
Figure 18. The Andes Mountains are a chain of continental arc volcanoes that build up as the Nazca Plate subducts beneath the South American Plate.
When tensional stresses pull crust apart, it breaks into blocks that slide up and drop down along normal faults. The result is alternating mountains and valleys, known as a basin-and-range (figure 19).
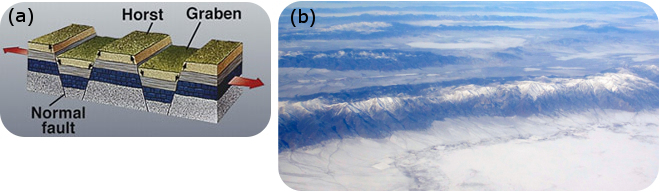
Figure 19. (a) In basin-and-range, some blocks are uplifted to form ranges, known as horsts, and some are down-dropped to form basins, known as grabens. (b) Mountains in Nevada are of classic basin-and-range form.
This is a very quick animation of movement of blocks in a basin-and-range setting.
Summary
- Stress is the force applied to a rock and may cause deformation. The three main types of stress are typical of the three types of plate boundaries: compression at convergent boundaries, tension at divergent boundaries, and shear at transform boundaries.
- Where rocks deform plastically, they tend to fold. Brittle deformation brings about fractures and faults.
- The two main types of faults are dip-slip (the fault plane is inclined to the horizontal) and strike-slip (the fault plane is perpendicular to the horizontal).
- The world’s largest mountains grow at convergent plate boundaries, primarily by thrust faulting and folding.
Strain
As we’ve just learned, the earth’s crust is constantly subjected to forces that push, pull, or twist it. These forces are called stress. In response to stress, the rocks of the earth undergo strain, also known as deformation.
Strain is any change in volume or shape.There are four general types of stress. One type of stress is uniform, which means the force applies equally on all sides of a body of rock. The other three types of stress, tension, compression and shear, are non-uniform, or directed, stresses.All rocks in the earth experience a uniform stress at all times. This uniform stress is called lithostatic pressure and it comes from the weight of rock above a given point in the earth. Lithostatic pressure is also called hydrostatic pressure. (Included in lithostatic pressure are the weight of the atmosphere and, if beneath an ocean or lake, the weight of the column of water above that point in the earth. However, compared to the pressure caused by the weight of rocks above, the amount of pressure due to the weight of water and air above a rock is negligible, except at the earth’s surface.) The only way for lithostatic pressure on a rock to change is for the rock’s depth within the earth to change.Because lithostatic pressure is a uniform stress, a change in lithostatic pressure does not cause fracturing and slippage along faults. Nevertheless, it may be the cause of certain types of earthquakes. In subducting tectonic plates, the increased pressure of greater depth within the earth may cause the minerals in the plate to metamorphose spontaneously into a new set of denser minerals that are stable at the higher pressure. This is thought to be the likely cause of certain types of deep earthquakes in subduction zones, including the deepest earthquakes ever recorded.
Rocks are also subjected to the three types of directed (non-uniform) stress – tension, compression, and shear.
- Tension is a directed (non-uniform) stress that pulls rock apart in opposite directions. The tensional (also called extensional) forces pull away from each other.
- Compression is a directed (non-uniform) stress that pushes rocks together. The compressional forces push towards each other.
- Shear is a directed (non-uniform) stress that pushes one side of a body of rock in one direction, and the opposite side of the body of rock in the opposite direction. The shear forces are pushing in opposite ways.
In response to stress, rock may undergo three different types of strain – elastic strain, ductile strain, or fracture.
- Elastic strain is reversible. Rock that has undergone only elastic strain will go back to its original shape if the stress is released.
- Ductile strain is irreversible. A rock that has undergone ductile strain will remain deformed even if the stress stops. Another term for ductile strain is plastic deformation.
- Fracture is also called rupture. A rock that has ruptured has abruptly broken into distinct pieces. If the pieces are offset—shifted in opposite directions from each other—the fracture is a fault.
Ductile and Brittle Strain
Earth’s rocks are composed of a variety of minerals and exist in a variety of conditions. In different situations, rocks may act either as ductile materials that are able to undergo an extensive amount of ductile strain in response to stress, or as brittle materials, which will only undergo a little or no ductile strain before they fracture. The factors that determine whether a rock is ductile or brittle include:
- Composition—Some minerals, such as quartz, tend to be brittle and are thus more likely to break under stress. Other minerals, such as calcite, clay, and mica, tend to be ductile and can undergo much plastic deformation. In addition, the presence of water in rock tends to make it more ductile and less brittle.
- Temperature—Rocks become softer (more ductile) at higher temperature. Rocks at mantle and core temperatures are ductile and will not fracture under the stresses that occur deep within the earth. The crust, and to some extent the lithosphere, are cold enough to fracture if the stress is high enough.
- Lithostatic pressure—The deeper in the earth a rock is, the higher the lithostatic pressure it is subjected to. High lithostatic pressure reduces the possibility of fracture because the high pressure closes fractures before they can form or spread. The high lithostatic pressures of the earth’s sub-lithospheric mantle and solid inner core, along with the high temperatures, are why there are no earthquakes deep in the earth.
- Strain rate—The faster a rock is being strained, the greater its chance of fracturing. Even brittle rocks and minerals, such as quartz, or a layer of cold basalt at the earth’s surface, can undergo ductile deformation if the strain rate is slow enough.
Most earthquakes occur in the earth’s crust. A smaller number of earthquakes occur in the uppermost mantle (to about 700 km deep) where subduction is taking place. Rocks in the deeper parts of the earth do not undergo fracturing and do not produce earthquakes because the temperatures and pressures there are high enough to make all strain ductile. No earthquakes originate from below the the earth’s upper mantle.
Stress and Fault Types
The following correlations can be made between types of stress in the earth, and the type of fault that is likely to result:
- Tension leads to normal faults.
- Compression leads to reverse or thrust faults.
- Horizontal shear leads to strike-slip faults.
Correlations between type of stress and type of fault can have exceptions. For example, zones of horizontal stress will likely have strike-slip faults as the predominant fault type. However there may be active normal and thrust faults in such zones as well, particularly where there are bends or gaps in the major strike-slip faults.
To give another example, in a region of compression stress in the crust, where sheets of rock are stacked on active thrust faults, strike-slip faults commonly connect some of the thrust faults together.
Check Your Understanding
Forces that act to elongate the crust are associated with ________.
- shear.
- tension.
- compression.
Show Answer
tension
Candela Citations
- Introduction to Stress and Strain. Authored by: Kimberly Schulte and Lumen Learning. Provided by: Lumen Learning. License: CC BY: Attribution
- 7.1: Stress in Earth's Crust. Provided by: CK-12. Located at: http://www.ck12.org/book/CK-12-Earth-Science-For-High-School/section/7.1/. License: CC BY-NC: Attribution-NonCommercial
- Basics -- Earthquakes. Authored by: Ralph L. Dawes and Cheryl D. Dawes. Provided by: Wenatchee Valley College. Located at: http://commons.wvc.edu/rdawes/G101OCL/Basics/earthquakes.html. Project: Geology 101 - Introduction to Physical Geology. License: CC BY: Attribution