Mountains
How do plate motions create mountains?
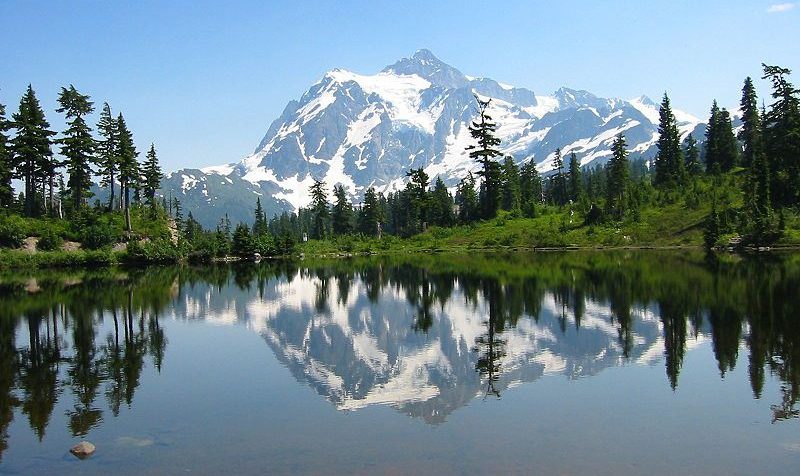
Plate tectonic processes create some of the world’s most beautiful places. The North Cascades Mountains in Washington State are a continental volcanic arc. The mountains currently host some glaciers and there are many features left by the more abundant ice age glaciers. Changes in altitude make the range a habitable place for many living organisms.
Converging Plates
Converging plates create the world’s largest mountain ranges. Each combination of plate types—continent-continent, continent-ocean, and ocean-ocean—creates mountains.
Converging Continental Plates
Some of the biggest mountain ranges in the world are created by the convergence of continental plates. The collision of the Indian sub-continent with Asia began with the subduction intervening oceanic crust beneath Asia, and significant generation of magma and “arc-volcanism” and volcanic mountains (much like today’s Cascade and Andes mountains), as per the section below (“subducting oceanic plates”). After the oceanic crust that sat between India and Asia was subducted, then India literally rammed into Asia and was pushed beneath.
Oceanic crust is easier to subduct than continental crust, largely because it is denser.
So when the continental crust of India was thrust beneath Asia, huge stresses developed from the collision and the subducted continental crust was buoyant enough to push the overlying mountains even higher!
See Figure 5, below.
This sort of collisional event and uplift will cause folds, reverse faults, and thrust faults, effectively raising and shortening the crust.
As noted previously there is currently no mountain range of this type in the western U.S., but at some point in the history of the Appalachian mountains (east part of U.S.), continental collision was involved and very likely quite high mountains!
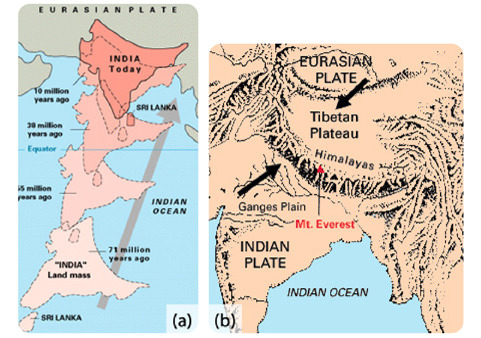
Figure 5. (a) The world’s highest mountain range, the Himalayas, is growing from the collision between the Indian and the Eurasian plates. (b) The crumpling of the Indian and Eurasian plates of continental crust creates the Himalayas.
Subducting Oceanic and Continental Plates
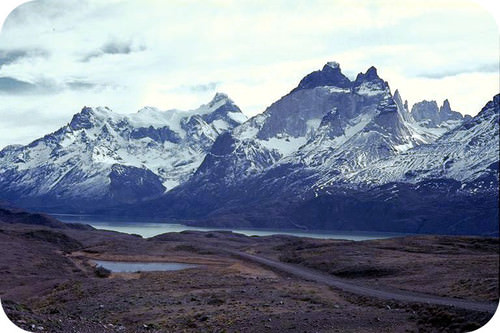
Figure 6. The Andes Mountains are a chain of continental arc volcanoes that build up as the Nazca Plate subducts beneath the South American Plate.
As with the pre-continent collision of India and Asia, oceanic crust can be subducted beneath continental crust. This, of course, is a subduction zone, and the ocean crust almost always is the subducted material, since it has a higher density.
Subduction of oceanic lithosphere beneath continental lithosphere,at convergent plate boundaries, also builds major mountain ranges.
Examples of this would include the Andes of South America (see Figure 6) and the Cascades of the U.S. Pacific Northwest region.
These mountains form as a result of both rising magma (above the subducted slab) and the compressional stresses of collision.
Subduction of Ocean Lithosphere beneath Ocean Lithosphere
Another collisional plate circumstance is that of oceanic lithosphere (and the crust that sits atop) being subducted beneath another plate of oceanic lithosphere.
This occurs in numerous places around the world, but a great example is that of the Aleutian Islands off Alaska.
It is a curved group of islands, and this feature is characteristic of ocean-ocean convergence, and ultimately results because of subducting slabs on a curved surface– i.e. the spherical shape of planet earth!
Because of the curvature, ocean-ocean convergence generates volcanic islands that are commonly referred to as volcanic arcs.
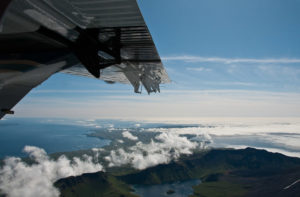
Kanaga Volcano, an Aleutian Arc volcano– a consequence of subduction of Pacific Plate beneath the Bering Sea Plate
Diverging Plates
Amazingly, even divergence can create mountain ranges. When tensional stresses pull crust apart, it breaks into blocks that slide up and drop down along normal faults. The result is alternating mountains and valleys, known as a basin-and-range (Figure 7). In basin-and-range, some blocks are uplifted to form ranges, known as horsts, and some are down-dropped to form basins, known as grabens.
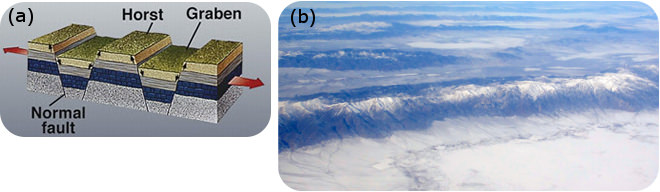
Figure 7. (a) Horsts and grabens. (b) Mountains in Nevada are of classic basin-and-range form.
The Western U.S. Mountains!
This is a digital elevation image of the United States. It shows NOTHING but elevation. It is color coded for elevation.
The most striking feature of this sort of map is that the east margin of the U.S. has some ripples, called the Appalachians, but the real action (at least in terms of elevated terrain!) is in the western U.S.
The Appalachians are a consequence of various mountain building events (called “orogenies”) that for the most part occurred during the Paleozoic, i.e. pre-dinosaur time, from about 500 million years ago to 250 million years ago.
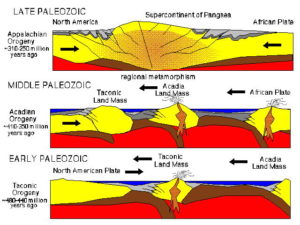
from Phil Stoffer and Paula Messina, Hunter College == http://www.geo.hunter.cuny.edu/bight/highland.html ==
In the adjacent image, we see that the Appalachians were all about various kinds of collisional tectonics. There was subduction of ocean crust under ocean crust, forming island arcs.
The island arcs were slammed up against proto-North-America during ocean-ocean convergence, and then the final phase of mountain building involved the collision of continental plates, namely North America and Africa, during the Late Paleozoic.
But, a very big idea here is that although there has been some degree of post-Paleozoic mountain building in the eastern U.S., it’s been largely a time of erosion and denudation. This is one reason why the Appalachians are relatively low in elevation compared to the western mountains of the U.S.
There is more to it though!
Seismic probing of the lithospheric mantle beneath the western U.S. suggests that relatively low density lithosphere exists beneath the Sierra Nevada Range, the Basin and Range region, and the Rocky Mountains. This low density, and relatively buoyant, lithosphere acts to “hold up” the overlying crust and allows for very high mountain elevations (compared to other parts of the U.S.).
The colorful image, on the right, of the U.S. is based on seismic wave velocities.
The image uses S-wave velocities in the upper-most mantle. This would be the lower part of the what we continental lithosphere (remember, lithosphere is mantle plus overlying crust), just beneath the crust.
Quite interestingly,
In this image we see a lot of “warmer” colors of red and yellow.in the western U.S., coincident with the high elevation terrain of the Colorado Plateau, the Rocky Mountains, and the Sierra Mountains. The red/yellow colors indicate SLOWER seismic velocities, and therefore lower density material. Of course, low density mantle material (although still rock!), is relatively buoyant and will try to rise up relative to surrounding mantle.
The POINT OF ALL THIS– is that the velocities suggest quite a bit of warmer and lower density upper mantle in the western U.S., and most likely, this warmer mantle is helping to sustain the high mountains and high overall terrain in this part of North America.
MAKING CONTINENTAL CRUST
How Do “Plates” of Continental-type FORM in the first place?
After all this discussion of plates bumping into each other (convergence) or getting pulled apart (divergence) or sliding past one another (transform), one might ask “How do Plates form?”
In fact, another good question would be– “The earth seems to be mostly covered by OCEANIC CRUST and less so by CONTINENTAL CRUST. Why is this the case?”
(Remember in elementary school— we learned that the earth is mostly covered by oceans… somewhere between 2/3 and 3/4 of earth surface.)
It turns out that Plate Tectonics is a key to answering this question.
The most “primary” sort of crust is oceanic crust. It forms when the deep mantle (asthenosphere) wells up at mid ocean ridges and generates NEW crust of the basaltic, or oceanic, variety.
Later, this basaltic crust SUBDUCTS, typically at the margins of big ocean basins (but not always). And where subduction occurs, NEW magma rises of a more granitic type, and this is how mountains and new continental crust is made.
In the diagram below, note how subducting ocean crust generates bubbles of magma that rises to make the (speckled/dotted) continental crust. As the intervening ocean crust is removed by subduction, larger and larger masses of continental crust form!
This is what we think was occurring during the earliest times of continental crust formation– in the Precambrian.
Age Provinces of North America
So, as per the above discussion on the formation of continental crust– we’d expect that early continents had new continental fragments added on with time.
This is exactly what we see in North America (and on many other continents)– the oldest continental crust is in the north part of the continent, in what we call the Canadian Shield region. In the adjoining diagram, the Canadian Shield is composed of very old rock (purple and pink), in excess of 2.3 billion years. Around this “proto-continent” we find younger and younger crust, e.g. the green and yellow and blue material, younger than 1 billion years.
To be clear– much of the old crust, or “basement rock” as it is sometimes called, can be covered by younger sediments. So the colored map here of North American Age Provinces refers to the deep roots of the continent. These roots are called the continental CRATON, and where it is exposed (with no overlying sediment of younger age) it is called a SHIELD region.
Summary
- Converging or diverging plates cause mountains to grow.
- Subduction of oceanic crust beneath a continental or oceanic plate creates a volcanic arc.
- Tensional forces bring about block faulting, which creates a basin-and-range topography
- All the above help to generate continental crust– primarily from sweeping together volcanic arcs and continent pieces.