Scientific Method– What is it and why bother?
Hard rock climbs involve falling. But technology makes these falls pretty safe– ropes that are strong yet light, expansion bolts that hold multi-thousand pound loads.
Of course, we live in a world where technology is everywhere– from contact lenses to smart phones to satellite gps (and radio!) and more.
And, sure sure– we gotta love science because it gives us all kinds of great medicines and technology. But I think there is more… much more!
We use a kind of scientific approach (rational, logical, testable) to all kinds of things– how we see law, economics, and perhaps even politics!
Furthermore, science gives us two big ideas… our world and universe are huge, and they are immensely old!
Scientific Process/Method is not only at the heart of geology and other scientific disciplines, but in many ways a key part of our culture.
If we think of science as beginning with IDEAS (hypotheses), followed by well substantiated EVIDENCE and TESTING, and eventually UNDERSTANDING (corroborated theory)– we have to recognize that this way of approaching problems is also at the root of business, economics, law, politics, and almost everything we do!
(In our culture, we try not to just “believe” whatever someone tells us. We “want the facts” and we want to understand what those facts imply!)
What You’ll Learn to Do
- View the Scientific Method as a Process
- Recognize the Scientific Method as more than a cookbook set of steps!
- Form a hypothesis and use it to design a scientific experiment.
Sometime in your life you’ve undoubtedly asked questions about the world around you. Perhaps you’ve wondered why the sky is blue and not some other color; or why we hear an echo across a big canyon; or why we have seasons or lunar phases. Questions about nature are certainly part of doing science, but contrary to some simplified explanations of science, it’s much more than just “asking questions.” In many ways, good science is all about asking the right questions! It’s about asking the questions whose answers really tell us more about how the world works.
At its essence, doing science involves wondering about how nature works, then coming up with an idea (hypothesis/theory), and then testing that idea, and re-working the idea till it fits observation, and finally achieving a model that explains certain natural phenomena.
Ever seen this sketch (by W.E. Hill)??
It can be seen as an old woman with a big pointed chin–we see her left side in profile.
It can also be seen as a young woman with dainty nose and a hint of her left eyelash; we see her left side, but her head is mostly turned away from us, as she is turned to the right.
Like this image, over the last 500 years, scientific revolutions have shown that a reigning perspective on the world is not the only way to see the world. If we look more carefully, it’s surprising what may be seen!
Along these same lines, here is a big idea: Science is NOT “proving things” by seeing them happen!
It’s NOT very easy to see sub-atomic particles, or the process of macroscopic organisms undergoing speciation, or 80 mile thick pieces of earth’s crust sliding around, but we’re pretty confident that we know a lot about these natural processes, and hence we have atomic theory, evolution, and plate tectonics..
In fact, science often shows us that the world is NOT at all how it looks!
Scientific Method
Ugh! You’ve probably seen this “flow chart” ever since elementary school.
Indeed, the scientific method can be thought of as a series of steps that help us to eventually elucidate the way the world works.
And, the steps do “flow” in a logical and rational manner. The “doing of science”– from wonder to ideas to testing– is rooted in a logical approach.
Here is a typical scientific method flow chart. See Figure 1.
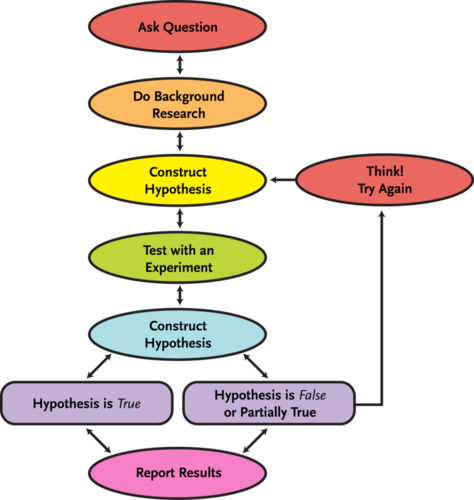
Figure 1. The Scientific Method
An Example of Scientific Reasoning and Process
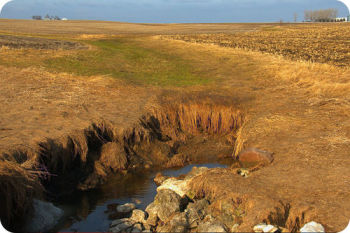
Figure 2. Soil is often lost from ground that has been plowed.
Let’s say a farmer is having an erosion problem; every year her farm is losing soil. The farmer learns that a farming method called “no-till farming” allows farmers to plant seeds without plowing the land. She wonders if planting seeds without plowing will reduce the erosion problem and help keep more soil on her farmland.
The process might begin with our farmer doing some research (books, journals, other farmers, etc.) as a means to investigate what causes erosion and how avoiding plowing might help. See Figure 2.
Hypothesis
Consider her hypothesis.
“If I don’t plow my fields, I will lose less soil than if I do plow the fields. Plowing disrupts the soil and breaks up roots that help hold soil in place. Furthermore, plowing generates flow paths for water that can carry away soil.” A hypothesis is a reasonable explanation that can be tested.
Experiment/Testing
When we design experiments, it’s best to keep things simple. Ideally, during the experiment we only change one aspect of the system–the independent variable.
In this example, the farmer chooses two fields and then changes only one thing between them. She changes how she plows her fields. One field will be tilled and one will not. Everything else (crops, fertilizer, amount of irrigation) will be the same on both fields. These are the experimental controls.
The farmer will measure how much soil is lost from each field. This is the dependent variable. How much soil is lost from each field “depends” on the plowing method.
Data and Experimental Error
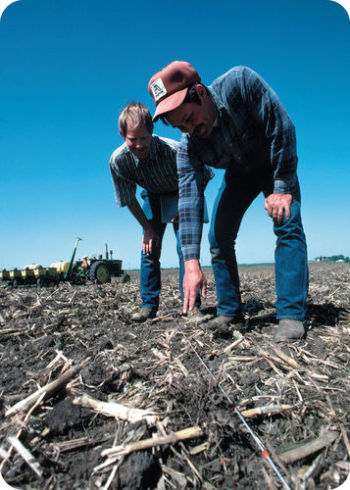
Figure 4. A pair of farmers take careful measurements in the field.
Experimental data involves making measurements using anything from a balance to a ruler to an electron microprobe.
Graphical representation of data is typically useful, comparing independent and dependent variables.
Good to keep in mind– With measurement, it’s crucial to understand the capability of our observations and devices. For example,when weighing something, if we find a difference of one gram between experimental “runs” and the balance is not capable of reproducing measurements better than plus/minus a gram or so, then that difference is really rather meaningless!
Scientists speak of precision, in essence meaning how many digits and decimal places we can record from an instrument. For example, I might measure a weight of soil as 2.569473204 grams, which is rather precise!
But scientists must also consider the likely accuracy of measurements, in essence how confident we can be with a particular measurement. For example, if my balance can only reproduce to about one half of a gram, then I should really only say that the soil is around 2-3 grams in weight (and not report any decimals)!
Conclusions
Drawing a conclusion requires evaluating the data and deciding how hypothesis fared. Perhaps the hypothesis requires modification (tweaking) and this should then involve more testing.
Perhaps after two seasons of crop growth, our farmer finds that 2.2 times as much soil was lost on the plowed field as compared to the un-plowed field. She concludes that her hypothesis was correct. The farmer also notices some other differences in the two plots. The plants in the no-till plots are taller. The soil moisture seems higher. She decides to repeat the experiment. This time she will measure soil moisture, plant growth, and the total amount of water the plants consume. It’s a good start, and from now on she will use no-till methods of farming.
Theory
In the process of growing up and becoming an adult, there’s really no exact point where you can say “Well, yesterday I was a kid, and today I am a full fledged adult”.
Similarly, there’s no one point where a good hypothesis becomes a full fledged and working theory!
Ultimately, enough scientists evaluate and test a hypothesis, and once it works well to explain the world (whether it’s the behavior of atoms, or light, or shifting continents, or “no-till” farming!) then it becomes a useful paradigm for interpreting and acquiring additional knowledge.
It’s worth noting that the assessment of how well a hypothesis works, prior to becoming a theory, is carefully scrutinized by other scientists. This is generally done via the publishing of papers in scientific journals. Important and trustworthy scientific journals are “peer-reviewed” meaning that other scientists will look over the work of those who are publishing. The reviewers are experts in the particular field at hand, and they evaluate the quality of the data and the experiments that have been conducted. If there are discrepancies, errors, or faulty reasoning, then the paper is denied publication. It’s a bit on the “rough” side, but scientists actually take some delight in finding problems with each others work! The good part is that this really makes science quite trustworthy; only very high quality work is allowed to published and become part of the bank of scientific knowledge.
Quantum theory, relativity theory, organic evolution theory, plate tectonic theory, etc etc etc., are incredibly well-tested theories, via literally tens of thousands of experiments and peer-reviewed scientific papers.
Are these well-established theories 100% correct and true and absolute explanations for the world around us? Definitely not! They are very good, but with time most theories are modified and re-worked. Science is not static; it constantly re-evaluates and improves its understanding of nature.