The sensory systems of touch and pain provide us with information about our environment and our bodies that is often crucial for survival and well-being. Moreover, touch is a source of pleasure. In this module, we review how information about our environment and our bodies is coded in the periphery and interpreted by the brain as touch and pain sensations. We discuss how these experiences are often dramatically shaped by top-down factors like motivation, expectation, mood, fear, stress, and context. When well-functioning, these circuits promote survival and prepare us to make adaptive decisions. Pathological loss of touch can result in perceived disconnection from the body, and insensitivity to pain can be very dangerous, leading to maladaptive hazardous behavior. On the other hand, chronic pain conditions, in which these systems start signaling pain in response to innocuous touch or even in the absence of any observable sensory stimuli, have tremendous negative impact on the lives of the affected. Understanding how our sensory-processing mechanisms can be modulated psychologically and physiologically promises to help researchers and clinicians find new ways to alleviate the suffering of chronic-pain patients
Introduction
Imagine a life free of pain. How would it be—calm, fearless, serene? Would you feel invulnerable, invincible? Getting rid of pain is a popular quest—a quick search for “pain-free life” on Google returns well over 4 million hits—including links to various bestselling self-help guides promising a pain-free life in only 7 steps, 6 weeks, or 3 minutes. Pain management is a billion-dollar market, and involves much more than just pharmaceuticals. Surely a life with no pain would be a better one?
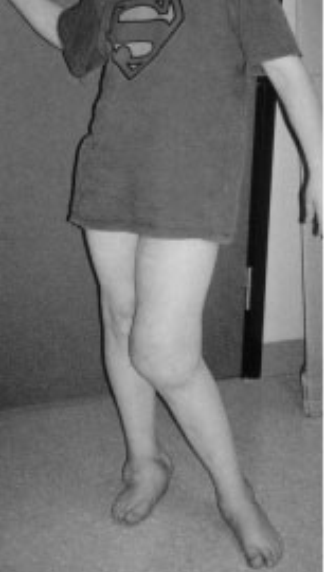
Well, consider one of the “lucky few”: 12-year-old “Thomas” has never felt deep pain. Not even when a fracture made him walk around with one leg shorter than the other, so that the bones of his healthy leg were slowly crushed to destruction underneath the knee joint (see Figure 1A). For Thomas and other members of a large Swedish family, life without pain is a harsh reality because of a mutated gene that affects the growth of the nerves conducting deep pain. Most of those affected suffer from joint damage and frequent fractures to bones in their feet and hands; some end up in wheelchairs even before they reach puberty (Minde et al., 2004). It turns out pain—generally—serves us well.
Living without a sense of touch sounds less attractive than being free of pain—touch is a source of pleasure and essential to how we feel. Losing the sense of touch has severe implications—something patient G. L. experienced when an antibiotics treatment damaged the type of nerves that signal touch from her skin and the position of her joints and muscles. She reported feeling like she’d lost her physical self from her nose down, making her “disembodied”—like she no longer had any connection to the body attached to her head. If she didn’t look at her arms and legs they could just “wander off” without her knowing—initially she was unable to walk, and even after she relearned this skill she was so dependent on her visual attention that closing her eyes would cause her to land in a hopeless heap on the floor. Only light caresses like those from her children’s hands can make her feel she has a body, but even these sensations remain vague and elusive (Olausson et al., 2002; Sacks, 1985).
Sensation
Cutaneous Senses of the Skin Connect the Brain to the Body and the Outside World
Touch and pain are aspects of the somatosensory system, which provides our brain with information about our own body (interoception) and properties of the immediate external world (exteroception) (Craig, 2002). We have somatosensory receptors located all over the body, from the surface of our skin to the depth of our joints. The information they send to the central nervous system is generally divided into four modalities: cutaneous senses (senses of the skin), proprioception (body position), kinesthesis (body movement), and nociception (pain, discomfort). We are going to focus on the cutaneous senses, which respond to tactile, thermal, and pruritic (itchy) stimuli, and events that cause tissue damage (and hence pain). In addition, there is growing evidence for a fifth modality specifically channeling pleasant touch (McGlone & Reilly, 2010).
Different Receptor Types Are Sensitive to Specific Stimuli
The skin can convey many sensations, such as the biting cold of a wind, the comfortable pressure of a hand holding yours, or the irritating itch from a woolen scarf. The different types of information activate specific receptors that convert the stimulation of the skin to electrical nerve impulses, a process called transduction. There are three main groups of receptors in our skin: mechanoreceptors, responding to mechanical stimuli, such as stroking, stretching, or vibration of the skin; thermoreceptors, responding to cold or hot temperatures; and chemoreceptors, responding to certain types of chemicals either applied externally or released within the skin (such as histamine from an inflammation). For an overview of the different receptor types and their properties, see Box 1. The experience of pain usually starts with activation of nociceptors—receptors that fire specifically to potentially tissue-damaging stimuli. Most of the nociceptors are subtypes of either chemoreceptors or mechanoreceptors. When tissue is damaged or inflamed, certain chemical substances are released from the cells, and these substances activate the chemosensitive nociceptors. Mechanoreceptive nociceptors have a high threshold for activation—they respond to mechanical stimulation that is so intense it might damage the tissue.
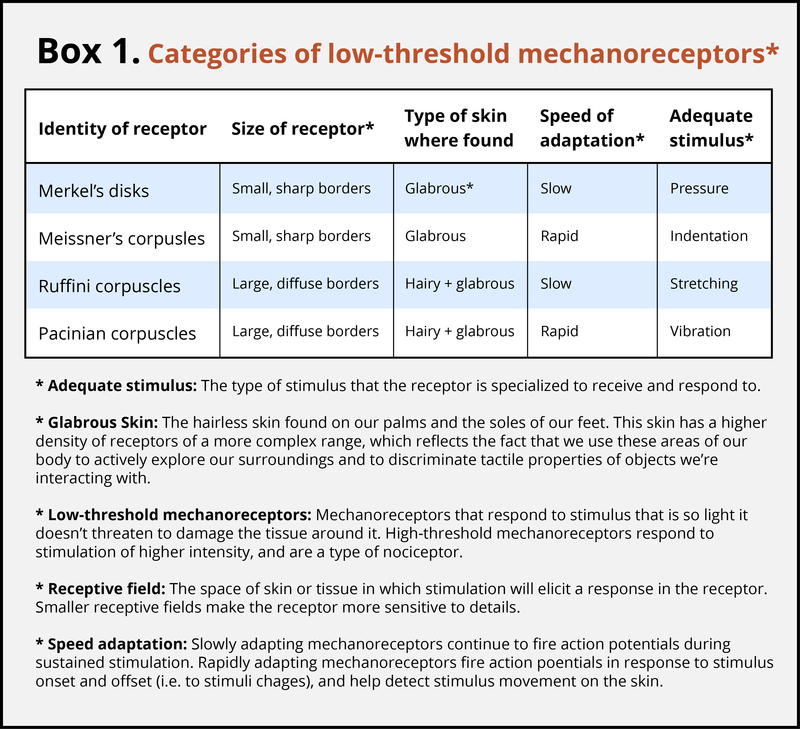
Action Potentials in the Receptor Cells Travel as Nerve Impulses with Different Speeds
When you step on a pin, this activates a host of mechanoreceptors, many of which are nociceptors. You may have noticed that the sensation changes over time. First you feel a sharp stab that propels you to remove your foot, and only then you feel a wave of more aching pain. The sharp stab is signaled via fast-conducting A-fibers, which project to the somatosensory cortex. This part of the cortex is somatotopically organized—that is, the sensory signals are represented according to where in the body they stem from (see llustration, Figure 2). The unpleasant ache you feel after the sharp pin stab is a separate, simultaneous signal sent from the nociceptors in your foot via thin C-pain or Aδ-fibers to the insular cortex and other brain regions involved in processing of emotion and interoception (see Figure 3a for a schematic representation of this pathway). The experience of stepping on a pin is, in other words, composed by two separate signals: one discriminatory signal allowing us to localize the touch stimulus and distinguish whether it’s a blunt or a sharp stab; and one affective signal that lets us know that stepping on the pin is bad. It is common to divide pain into sensory–discriminatory and affective–motivational aspects (Auvray, Myin, & Spence, 2010). This distinction corresponds, at least partly, to how this information travels from the peripheral to the central nervous system and how it is processed in the brain (Price, 2000).
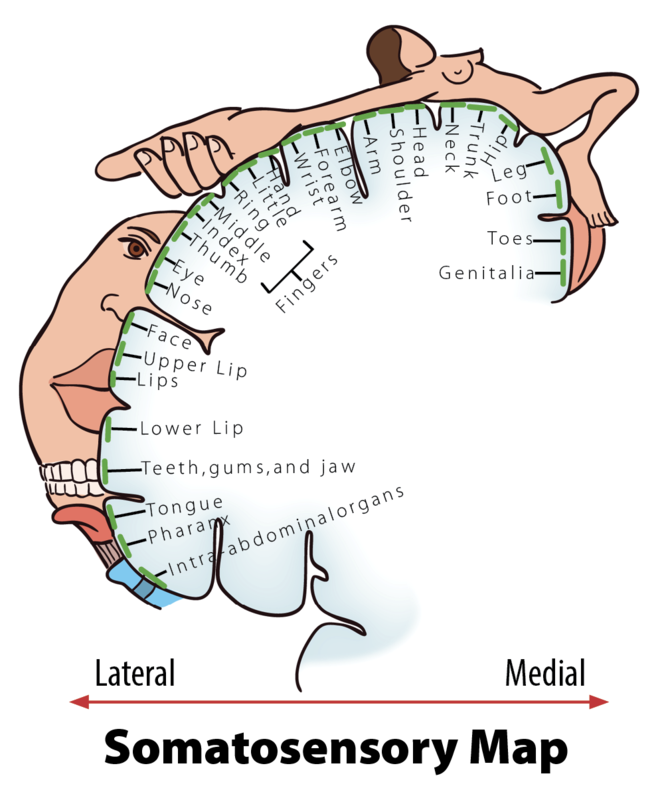
Affective Aspects of Touch Are Important for Development and Relationships
Touch senses are not just there for discrimination or detection of potentially painful events, as Harlow and Suomi (1970) demonstrated in a series of heartbreaking experiments where baby monkeys were taken from their mothers. The infant monkeys could choose between two artificial surrogate mothers—one “warm” mother without food but with a furry, soft cover; and one cold, steel mother with food. The monkey babies spent most of their time clinging to the soft mother, and only briefly moved over to the hard, steel mother to feed, indicating that touch is of “overpowering importance” to the infant (Harlow & Suomi, 1970, p. 161). Gentle touch is central for creating and maintaining social relationships in primates; they groom each other by stroking the fur and removing parasites—an activity important not only for their individual well-being but also for group cohesion (Dunbar, 2010; Keverne, Martensz, & Tuite, 1989). Although people don’t groom each other in the same way, gentle touch is important for us, too.
The sense of touch is the first to develop while one is in the womb, and human infants crave touch from the moment they’re born. From studies of human orphans, we know that touch is also crucial for human development. In Romanian orphanages where the babies were fed but not given regular attention or physical contact, the children suffered cognitive and neurodevelopmental delay (Simons & Land, 1987). Physical contact helps a crying baby calm down, and the soothing touch a mother gives to her child is thought to reduce the levels of stress hormones such as cortisol. High levels of cortisol have negative effects on neural development, and they can even lead to cell loss (Feldman, Singer, & Zagoory, 2010; Fleming, O’Day, & Kraemer, 1999; Pechtel & Pizzagalli, 2011). Thus, stress reduction through hugs and caresses might be important not only for children’s well-being, but also for the development of the infant brain.
The skin senses are similar across species, likely reflecting the evolutionary advantage of being able to tell what is touching you, where it’s happening, and whether or not it’s likely to cause tissue damage. An intriguing line of touch research suggests that humans, cats, and other animals have a special, evolutionarily preserved system that promotes gentle touch because it carries social and emotional significance.On a peripheral level, this system consists of a subtype of C-fibers that responds not to painful stimuli, but rather to gentle stroking touch—called C-tactile fibers. The firing rate of the C-tactile fibers correlates closely with how pleasant the stroking feels—suggesting they are coding specifically for the gentle caresses typical of social affiliative touch (Löken, Wessberg, Morrison, McGlone, & Olausson, 2009). This finding has led to the social touch hypothesis, which proposes that C-tactile fibers form a system for touch perception that supports social bonding (Morrison, Löken, & Olausson, 2010; Olausson, Wessberg, Morrison, McGlone, & Vallbo, 2010). The discovery of the C-tactile system suggests that touch is organized in a similar way to pain; fast-conducting A-fibers contribute to sensory–discriminatory aspects, while thin C-fibers contribute to affective–motivational aspects (Löken, Wessberg, Morrison, McGlone, & Olausson, 2009). However, while these “hard-wired” afferent systems often provide us with accurate information about our environment and our bodies, how we experience touch or pain depends very much on top-down sources like motivation, expectation, mood, fear, and stress.
Modulation
Pain Is Necessary for Survival, but Our Brain Can Stop It if It Needs To
In April 2003, the climber Aron Ralston found himself at the floor of Blue John Canyon in Utah, forced to make an appalling choice: face a slow but certain death—or amputate his right arm. Five days earlier he fell down the canyon—since then he had been stuck with his right arm trapped between an 800-lb boulder and the steep sandstone wall. Weak from lack of food and water and close to giving up, it occurred to him like an epiphany that if he broke the two bones in his forearm he could manage to cut off the rest with his pocket knife. The thought of freeing himself and surviving made him so exited he spent the next 40 minutes completely engrossed in the task: first snapping his bones using his body as a lever, then sticking his fingers into the arm, pinching bundles of muscle fibers and severing them one by one, before cutting the blue arteries and the pale “noodle-like” nerves. The pain was unimportant. Only cutting through the thick white main nerve made him stop for a minute—the flood of pain, he describes, was like thrusting his entire arm “into a cauldron of magma.” Finally free, he rappelled down a cliff and walked another 7 miles until he was rescued by some hikers (Ralston, 2010). How is it possible to do something so excruciatingly painful to yourself, and still manage to walk, talk, and think rationally afterwards? The answer lies within the brain, where signals from the body are interpreted. When we perceive somatosensory and nociceptive signals from the body, the experience is highly subjective and malleable by motivation, attention, emotion, and context.
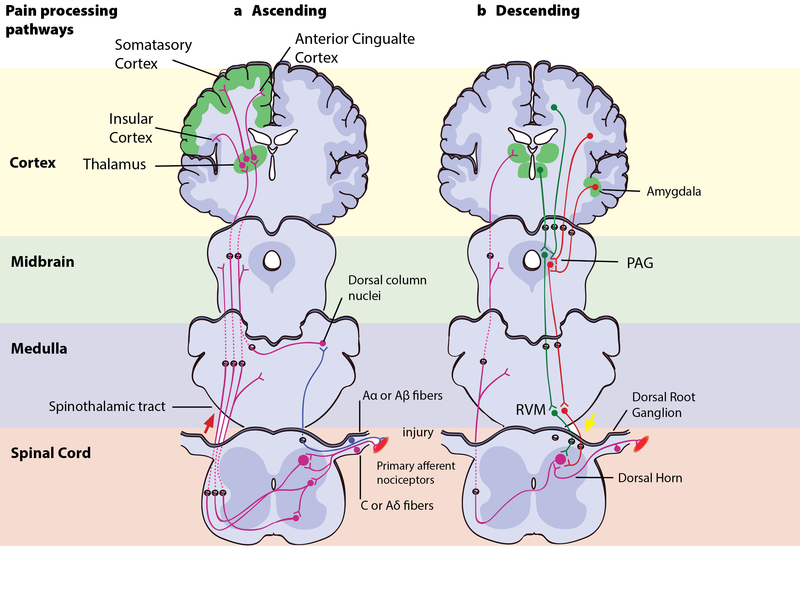
Right – Descending pain modulation pathways: Information from the environment and certain motivational states can activate this top–down pathway. Several areas in the limbic forebrain including the anterior cingulate and insular cortex, nuclei in the amygdala and the hypothalamus (H), project to the midbrain periaqueductal grey (PAG), which then modulates ascending pain transmission from the afferent pain system indirectly through the rostral ventromedial medulla (RVM) in the brainstem. This modulating system produces analgesia by the release of endogenous opioids, and uses ON- and OFF-cells to exert either inhibitory (green) or facilitatory (red) control of nociceptive signals at the spinal dorsal horn.
The Motivation–Decision Model and Descending Modulation of Pain
According to the motivation–decision model, the brain automatically and continuously evaluates the pros and cons of any situation—weighing impending threats and available rewards (Fields, 2004, 2006). Anything more important for survival than avoiding the pain activates the brain’s descending pain modulatory system—a top-down system involving several parts of the brain and brainstem, which inhibits nociceptive signaling so that the more important actions can be attended to (Figure 3b). In Aron’s extreme case, his actions were likely based on such an unconscious decision process—taking into account his homeostatic state (his hunger, thirst, the inflammation and decay of his crushed hand slowly affecting the rest of his body), the sensory input available (the sweet smell of his dissolving skin, the silence around him indicating his solitude), and his knowledge about the threats facing him (death, or excruciating pain that won’t kill him) versus the potential rewards (survival, seeing his family again). Aron’s story illustrates the evolutionary advantage to being able to shut off pain: The descending pain modulatory system allows us to go through with potentially life-saving actions. However, when one has reached safety or obtained the reward, healing is more important. The very same descending system can then “crank up” nociception from the body to promote healing and motivate us to avoid potentially painful actions. To facilitate or inhibit nociceptive signals from the body, the descending pain modulatory system uses a set of ON- or OFF-cells in the brainstem, which regulates how much of the nociceptive signal reaches the brain. The descending system is dependent on opioid signaling, and analgesics like morphine relieve pain via this circuit (Petrovic, Kalso, Petersson, & Ingvar, 2002).
The Analgesic Power of Reward
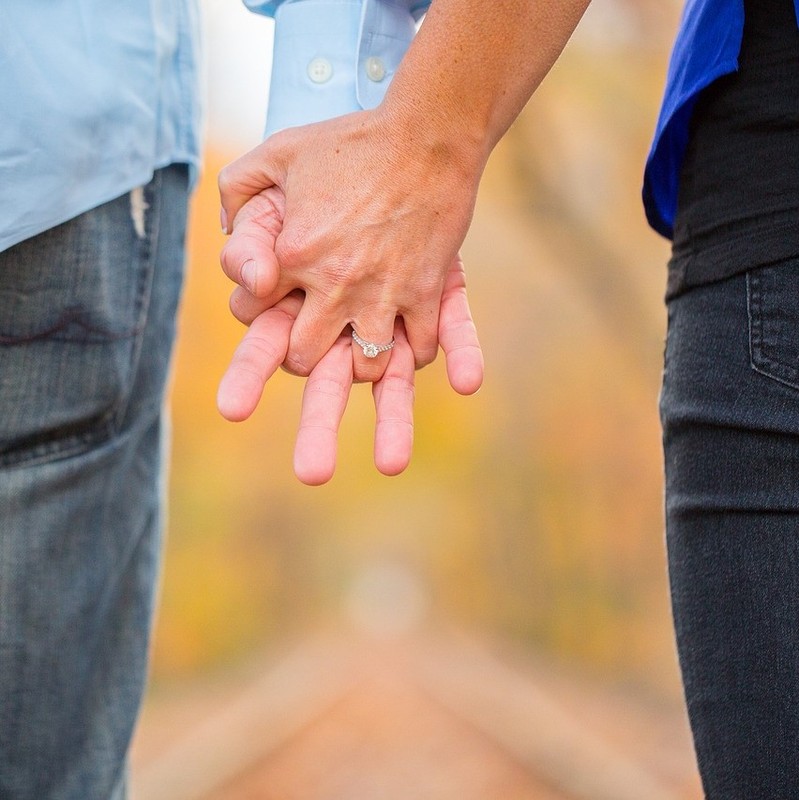
Thinking about the good things, like his loved ones and the life ahead of him, was probably pivotal to Aron’s survival. The promise of a reward can be enough to relieve pain. Expecting pain relief (getting less pain is often the best possible outcome if you’re in pain, i.e., it is a reward) from a medical treatment contributes to the placebo effect—where pain relief is due at least partly to your brain’s descending modulation circuit, and such relief depends on the brain’s own opioid system (Eippert et al., 2009; Eippert, Finsterbusch, Bingel, & Buchel, 2009; Levine, Gordon, & Fields, 1978). Eating tasty food, listening to good music, or feeling pleasant touch on your skin also decreases pain in both animals and humans, presumably through the same mechanism in the brain (Leknes & Tracey, 2008). In a now classic experiment, Dum and Herz (1984) either fed rats normal rat food or let them feast on highly rewarding chocolate-covered candy (rats love sweets) while standing on a metal plate until they learned exactly what to expect when placed there. When the plate was heated up to a noxious/painful level, the rats that expected candy endured the temperature for twice as long as the rats expecting normal chow. Moreover, this effect was completely abolished when the rats’ opioid (endorphin) system was blocked with a drug, indicating that the analgesic effect of reward anticipation was caused by endorphin release.
For Aron the climber, both the stress from knowing that death was impending and the anticipation of the reward it would be to survive probably flooded his brain with endorphins, contributing to the wave of excitement and euphoria he experienced while he carried out the amputation “like a five-year-old unleashed on his Christmas presents” (Ralston, 2010). This altered his experience of the pain from the extreme tissue damage he was causing and enabled him to focus on freeing himself. Our brain, it turns out, can modulate the perception of how unpleasant pain is, while still retaining the ability to experience the intensity of the sensation (Rainville, Duncan, Price, Carrier, & Bushnell, 1997; Rainville, Feine, Bushnell, & Duncan, 1992). Social rewards, like holding the hand of your boyfriend or girlfriend, have pain-reducing effects. Even looking at a picture of him/her can have similar effects—in fact, seeing a picture of a person we feel close to not only reduces subjective pain ratings, but also the activity in pain-related brain areas (Eisenberger et al., 2011). The most common things to do when wanting to help someone through a painful experience—being present and holding the person’s hand—thus seems to have a measurably positive effect.
When Touch Becomes Painful or Pain Becomes Chronic
Chances are you’ve been sunburned a few times in your life and have experienced how even the lightest pat on the back or the softest clothes can feel painful on your over-sensitive skin. This condition, where innocuous touch gives a burning, tender sensation, is similar to a chronic condition called allodynia—where neuronal disease or injury makes touch that is normally pleasant feel unpleasantly painful. In allodynia, neuronal injury in the spinal dorsal horn causes Aβ-afferents, which are activated by non-nociceptive touch, to access nociceptive pathways (Liljencrantz et al., 2013). The result is that even gentle touch is interpreted by the brain as painful. While an acute pain response to noxious stimuli has a vital protective function, allodynia and other chronic pain conditions constitute a tremendous source of unnecessary suffering that affects millions of people. Approximately 100 million Americans suffer from chronic pain, and annual economic cost associated is estimated to be $560–$635 billion (Committee on Advancing Pain Research, Care, & Institute of Medicine, 2011). Chronic pain conditions are highly diverse, and they can involve changes on peripheral, spinal, central, and psychological levels. The mechanisms are far from fully understood, and developing appropriate treatment remains a huge challenge for pain researchers.
Chronic pain conditions often begin with an injury to a peripheral nerve or the tissue surrounding it, releasing hormones and inflammatory molecules that sensitize nociceptors. This makes the nerve and neighboring afferents more excitable, so that also uninjured nerves become hyperexcitable and contribute to the persistence of pain. An injury might also make neurons fire nonstop regardless of external stimuli, providing near-constant input to the pain system. Sensitization can also happen in the brain and in the descending modulatory system of the brainstem (Zambreanu, Wise, Brooks, Iannetti, & Tracey, 2005). Exactly on which levels the pain perception is altered in chronic pain patients can be extremely difficult to pinpoint, making treatment an often exhausting process of trial and error. Suffering from chronic pain has dramatic impacts on the lives of the afflicted. Being in pain over a longer time can lead to depression, anxiety (fear or anticipation of future pain), and immobilization, all of which may in turn exacerbate pain (Wiech & Tracey, 2009). Negative emotion and attention to pain can increase sensitization to pain, possibly by keeping the descending pain modulatory system in facilitation mode. Distraction is therefore a commonly used technique in hospitals where patients have to undergo painful treatments like changing bandages on large burns. For chronic pain patients, however, diverting attention is not a long-term solution. Positive factors like social support can reduce the risk of chronic pain after an injury, and so they can help to adjust to bodily change as a result of injury. We haveve already talked about how having a hand to hold might alleviate suffering. Chronic pain treatment should target these emotional and social factors as well as the physiological.
The Power of the Mind
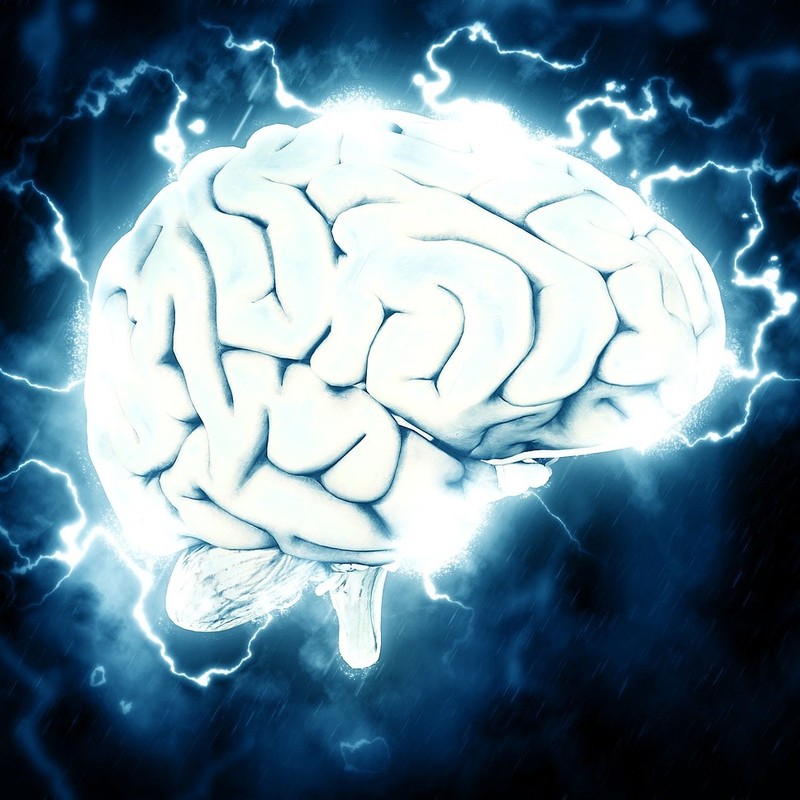
The context of pain and touch has a great impact on how we interpret it. Just imagine how different it would feel to Aron if someone amputated his hand against his will and for no discernible reason. Prolonged pain from injuries can be easier to bear if the incident causing them provides a positive context—like a war wound that testifies to a soldier’s courage and commitment—or phantom pain from a hand that was cut off to enable life to carry on. The relative meaning of pain is illustrated by a recent experiment, where the same moderately painful heat was administered to participants in two different contexts—one control context where the alternative was a nonpainful heat; and another where the alternative was an intensely painful heat. In the control context, where the moderate heat was the least preferable outcome, it was (unsurprisingly) rated as painful. In the other context it was the best possible outcome, and here the exact same moderately painful heat was actually rated as pleasant—because it meant the intensely painful heat had been avoided. This somewhat surprising change in perception—where pain becomes pleasant because it represents relief from something worse—highlights the importance of the meaning individuals ascribe to their pain, which can have decisive effects in pain treatment (Leknes et al., 2013). In the case of touch, knowing who or what is stroking your skin can make all the difference—try thinking about slugs the next time someone strokes your skin if you want an illustration of this point. In a recent study, a group of heterosexual males were told that they were about to receive sensual caresses on the leg by either a male experimenter or by an attractive female experimenter (Gazzola et al., 2012). The study participants could not see who was touching them. Although it was always the female experimenter who performed the caress, the heterosexual males rated the otherwise pleasant sensual caresses as clearly unpleasant when they believed the male experimenter did it. Moreover, brain responses to the “male touch” in somatosensory cortex were reduced, exemplifying how top-down regulation of touch resembles top-down pain inhibition.
Pain and pleasure not only share modulatory systems—another common attribute is that we don’t need to be on the receiving end of it ourselves in order to experience it. How did you feel when you read about Aron cutting through his own tissue, or “Thomas” destroying his own bones unknowingly? Did you cringe? It’s quite likely that some of your brain areas processing affective aspects of pain were active even though the nociceptors in your skin and deep tissue were not firing. Pain can be experienced vicariously, as can itch, pleasurable touch, and other sensations. Tania Singer and her colleagues found in an fMRI study that some of the same brain areas that were active when participants felt pain on their own skin (anterior cingulate and insula) were also active when they were given a signal that a loved one was feeling the pain. Those who were most “empathetic” also showed the largest brain responses (Singer et al., 2004). A similar effect has been found for pleasurable touch: The posterior insula of participants watching videos of someone else’s arm being gently stroked shows the same activation as if they were receiving the touch themselves (Morrison, Bjornsdotter, & Olausson, 2011).
Summary
Sensory experiences connect us to the people around us, to the rest of the world, and to our own bodies. Pleasant or unpleasant, they’re part of being human. In this module, we have seen how being able to inhibit pain responses is central to our survival—and in cases like that of climber Aron Ralston, that ability can allow us to do extreme things. We have also seen how important the ability to feel pain is to our health—illustrated by young “Thomas,” who keeps injuring himself because he simply doesn’t notice pain. While “Thomas” has to learn to avoid harmful activities without the sensory input that normally guides us, G. L. has had to learn how to keep approaching and move about in a world she can hardly feel at all, with a body that is practically disconnected from her awareness. Too little sensation or too much of it leads to no good, no matter how pleasant or unpleasant the sensation usually feels. As long as we have nervous systems that function normally, we are able to adjust the volume of the sensory signals and our behavioral reactions according to the context we’re in. When it comes to sensory signals like touch and pain, we are interpreters, not measuring instruments. The quest for understanding how our sensory–processing mechanisms can be modulated, psychologically and physiologically, promises to help researchers and clinicians find new ways to alleviate distress from chronic pain.
dig deeper
Web: Homepage for the International Association for the Study of Painhttp://www.iasp-pain.org
Web: Proceedings of the National Academy of Sciences Colloquium “The Neurobiology of Pain”http://www.pnas.org/content/96/14.toc#COLLOQUIUM
Web: Stanford School of Medicine Pain Management Centerhttp://paincenter.stanford.edu/
Website resource aiming to communicate “advances and issues in the clinical sciences as they relate to the role of the brain and mind in chronic pain disorders,” led by Dr. Lorimer Moseleyhttp://www.bodyinmind.org/
watch
Key Takeaways
- A-fibers
- Fast-conducting sensory nerves with myelinated axons. Larger diameter and thicker myelin sheaths increases conduction speed. Aβ-fibers conduct touch signals from low-threshold mechanoreceptors with a velocity of 80 m/s and a diameter of 10 μm; Aδ-fibers have a diameter of 2.5 μm and conduct cold, noxious, and thermal signals at 12 m/s. The third and fastest conducting A-fiber is the Aα, which conducts proprioceptive information with a velocity of 120 m/s and a diameter of 20 μm.
- Allodynia
- Pain due to a stimulus that does not normally provoke pain, e.g., when a light, stroking touch feels painful.
- Analgesia
- Pain relief.
- C-fibers
- C-fibers: Slow-conducting unmyelinated thin sensory afferents with a diameter of 1 μm and a conduction velocity of approximately 1 m/s. C-pain fibers convey noxious, thermal, and heat signals; C-tactile fibers convey gentle touch, light stroking.
- Chronic pain
- Persistent or recurrent pain, beyond usual course of acute illness or injury; sometimes present without observable tissue damage or clear cause.
- C-pain or Aδ-fibers
- C-pain fibers convey noxious, thermal, and heat signals
- C-tactile fibers
- C-tactile fibers convey gentle touch, light stroking
- Cutaneous senses
- The senses of the skin: tactile, thermal, pruritic (itchy), painful, and pleasant.
- Descending pain modulatory system
- A top-down pain-modulating system able to inhibit or facilitate pain. The pathway produces analgesia by the release of endogenous opioids. Several brain structures and nuclei are part of this circuit, such as the frontal lobe areas of the anterior cingulate cortex, orbitofrontal cortex, and insular cortex; and nuclei in the amygdala and the hypothalamus, which all project to a structure in the midbrain called the periaqueductal grey (PAG). The PAG then controls ascending pain transmission from the afferent pain system indirectly through the rostral ventromedial medulla (RVM) in the brainstem, which uses ON- and OFF-cells to inhibit or facilitate nociceptive signals at the spinal dorsal horn.
- Endorphin
- An endogenous morphine-like peptide that binds to the opioid receptors in the brain and body; synthesized in the body’s nervous system.
- Exteroception
- The sense of the external world, of all stimulation originating from outside our own bodies.
- Interoception
- The sense of the physiological state of the body. Hunger, thirst, temperature, pain, and other sensations relevant to homeostasis. Visceral input such as heart rate, blood pressure, and digestive activity give rise to an experience of the body’s internal states and physiological reactions to external stimulation. This experience has been described as a representation of “the material me,” and it is hypothesized to be the foundation of subjective feelings, emotion, and self-awareness.
- Nociception
- The neural process of encoding noxious stimuli, the sensory input from nociceptors. Not necessarily painful, and crucially not necessary for the experience of pain.
- Nociceptors
- High-threshold sensory receptors of the peripheral somatosensory nervous system that are capable of transducing and encoding noxious stimuli. Nociceptors send information about actual or impending tissue damage to the brain. These signals can often lead to pain, but nociception and pain are not the same.
- Noxious stimulus
- A stimulus that is damaging or threatens damage to normal tissues.
- Ocial touch hypothesis
- Proposes that social touch is a distinct domain of touch. C-tactile afferents form a special pathway that distinguishes social touch from other types of touch by selectively firing in response to touch of social-affective relevance; thus sending affective information parallel to the discriminatory information from the Aβ-fibers. In this way, the socially relevant touch stands out from the rest as having special positive emotional value and is processed further in affect-related brain areas such as the insula.
- Pain
- Defined as “an unpleasant sensory and emotional experience associated with actual or potential tissue damage, or described in terms of such damage,” according to the International Association for the Study of Pain.
- Phantom pain
- Pain that appears to originate in an amputated limb.
- Placebo effect
- Effects from a treatment that are not caused by the physical properties of a treatment but by the meaning ascribed to it. These effects reflect the brain’s own activation of modulatory systems, which is triggered by positive expectation or desire for a successful treatment. Placebo analgesia is the most well-studied placebo effect and has been shown to depend, to a large degree, on opioid mechanisms. Placebo analgesia can be reversed by the pharmacological blocking of opioid receptors. The word “placebo” is probably derived from the Latin word “placebit” (“it will please”).
- Sensitization
- Increased responsiveness of nociceptive neurons to their normal input and/or recruitment of a response to normally subthreshold inputs. Clinically, sensitization may only be inferred indirectly from phenomena such as hyperalgesia or allodynia. Sensitization can occur in the central nervous system (central sensitization) or in the periphery (peripheral sensitization).
- Social touch hypothesis
- Proposes that social touch is a distinct domain of touch. C-tactile afferents form a special pathway that distinguishes social touch from other types of touch by selectively firing in response to touch of social-affective relevance; thus sending affective information parallel to the discriminatory information from the Aβ-fibers. In this way, the socially relevant touch stands out from the rest as having special positive emotional value and is processed further in affect-related brain areas such as the insula.
- Somatosensory cortex
- Consists of primary sensory cortex (S1) in the postcentral gyrus in the parietal lobes and secondary somatosensory cortex (S2), which is defined functionally and found in the upper bank of the lateral sulcus, called the parietal operculum. Somatosensory cortex also includes parts of the insular cortex.
- Somatotopically organized
- When the parts of the body that are represented in a particular brain region are organized topographically according to their physical location in the body (see Figure 2 illustration).
- Spinothalamic tract
- Runs through the spinal cord’s lateral column up to the thalamus. C-fibers enter the dorsal horn of the spinal cord and form a synapse with a neuron that then crosses over to the lateral column and becomes part of the spinothalamic tract.
- Transduction
- The mechanisms that convert stimuli into electrical signals that can be transmitted and processed by the nervous system. Physical or chemical stimulation creates action potentials in a receptor cell in the peripheral nervous system, which is then conducted along the axon to the central nervous system.