What is the Immune System?
The immune system is a host defense system. It comprises many biological structures —ranging from individual white blood cells to entire organs — as well as many complex biological processes. The function of the immune system is to protect the host from pathogens and other causes of disease such as tumor cells. To function properly, the immune system must be able to detect a wide variety of pathogens. It also must be able to distinguish the cells of pathogens from the host’s own cells and also to distinguish cancerous or damaged host cells from healthy cells. In humans and most other vertebrates, the immune system consists of layered defenses that have increased specificity for particular pathogens or tumor cells. The layered defenses of the human immune system are usually classified into two subsystems called the innate immune system and the adaptive immune system.
Innate Immune System

The innate immune system provides a very quick but non-specific response to pathogens. It responds the same way regardless of the type of pathogen that is attacking the host. It includes barriers — such as the skin and mucous membranes — that normally keep pathogens out of the body. It also includes general responses to pathogens that manage to breach these barriers, including chemicals and cells that attack the pathogens inside the human host. For example, certain leukocytes (white blood cells) engulf and destroy pathogens they encounter in the process called phagocytosis, which is illustrated in Figure 20.2.220.2.2. Exposure to pathogens leads to an immediate maximal response from the innate immune system.
Adaptive Immune System
The adaptive immune system is activated if pathogens successfully enter the body and manage to evade the general defenses of the innate immune system. An adaptive response is specific to the particular type of pathogen that has invaded the body or to cancerous cells. It takes longer to launch a specific attack, but once it is underway, its specificity makes it very effective. An adaptive response also usually leads to immunity. This is a state of resistance to a specific pathogen due to the ability of the adaptive immune system to “remember” the pathogen and immediately mount a strong attack tailored to that particular pathogen if it invades again in the future.
Self vs. Non-Self
Both innate and adaptive immune responses depend on the ability of the immune system to distinguish between self and non-self molecules. Self molecules are those components of an organism’s body that can be distinguished from foreign substances by the immune system. Virtually all body cells have surface proteins that are part of a complex called the major histocompatibility complex (MHC). These proteins are one way the immune system recognizes body cells as self. Non-self proteins, in contrast, are recognized as foreign because they are different from self-proteins.
Antigens and Antibodies
Many non-self molecules comprise a class of compounds called antigens. Antigens, which are usually proteins, bind to specific receptors on immune system cells and elicit an adaptive immune response. Some adaptive immune system cells (B cells) respond to foreign antigens by producing antibodies. An antibody is a molecule that precisely matches and binds to a specific antigen. This may target the antigen (and the pathogen displaying it) for destruction by other immune cells.
Antigens on the surface of pathogens are how the adaptive immune system recognizes specific pathogens. Antigen specificity allows for the generation of responses tailored to the specific pathogen. It is also how the adaptive immune system ”remembers” the same pathogen in the future.
Immune Surveillance
Another important role of the immune system is to identify and eliminate tumor cells. This is called immune surveillance. The transformed cells of tumors express antigens that are not found on normal body cells. The main response of the immune system to tumor cells is to destroy them. This is carried out primarily by aptly named killer T cells of the adaptive immune system.
Lymphatic System
The lymphatic system is a human organ system that is a vital part of the adaptive immune system. It is also part of the cardiovascular system and plays a major role in the digestive system (see the concept Lymphatic System). The major structures of the lymphatic system are shown in Figure 20.2.320.2.3.
The lymphatic system consists of several lymphatic organs and a body-wide network of lymphatic vessels that transport the fluid called lymph. Lymph is essentially blood plasma that has leaked from capillaries into tissue spaces. It includes many leukocytes, especially lymphocytes, which are the major cells of the lymphatic system. Like other leukocytes, lymphocytes defend the body. There are several different types of lymphocytes that fight pathogens or cancer cells as part of the adaptive immune system.
Major lymphatic organs include the thymus and bone marrow. Their function is to form and/or mature lymphocytes. Other lymphatic organs include the spleen, tonsils, and lymph nodes, which are small clumps of lymphoid tissue clustered along lymphatic vessels. These other lymphatic organs harbor mature lymphocytes and filter lymph. They are sites where pathogens collect and adaptive immune responses generally begin.

Neuroimmune System vs. Peripheral Immune System
The brain and spinal cord are normally protected from pathogens in the blood by the selectively permeable blood-brain and blood-spinal cord barriers. These barriers are part of the neuroimmune system. The neuroimmune system has traditionally been considered to be distinct from the rest of the immune system, which is called the peripheral immune system, although that view may be changing (see the following Feature: Human Biology in the News). Unlike the peripheral system, in which leukocytes are the main cells, the main cells of the neuroimmune system are thought to be nervous system cells called glial cells. These cells are able to recognize and respond to pathogens, debris, and other potential dangers. Types of glial cells involved in neuroimmune responses include microglial cells and astrocytes.
- Microglial cells are among the most prominent types of glial cells in the brain. One of their main functions is to phagocytize cellular debris that remains when neurons die. Microglial cells also “prune” obsolete synapses between neurons.
- Astrocytes are glial cells that have different immune functions. They allow certain immune cells from the peripheral immune system to cross the blood-brain barrier into the brain to target both pathogens and damaged nervous tissue.
Innate Immune System
The innate immune system is a subset of the human immune system that produces rapid but non-specific responses to pathogens. Innate responses are generic rather than tailored to a particular pathogen. Every pathogen that is encountered is responded to in the same general ways by the innate system. Although the innate immune system provides immediate and rapid defenses against pathogens, it does not confer long-lasting immunity to them. In most organisms, the innate immune system is the dominant system of host defense. Other than most vertebrates including humans, the innate immune system is the only system of host defense.
In humans, the innate immune system includes surface barriers, inflammation, the complement system, and a variety of cellular responses. Surface barriers of various types generally keep most pathogens out of the body. If these barriers fail, then other innate defenses are triggered. The triggering event is usually the identification of pathogens by pattern-recognition receptors on cells of the innate immune system. These receptors recognize molecules that are broadly shared by pathogens but distinguishable from host molecules. Alternatively, the other innate defenses may be triggered when damaged, injured, or stressed cells send out alarm signals, many of which are recognized by the same receptors as those that recognize pathogens.
Barriers to Pathogens
The body’s first line of defense consists of three different types of barriers that keep most pathogens out of body tissues. The types of barriers are mechanical, chemical, and biological barriers.
Mechanical Barriers
Mechanical barriers are the first line of defense against pathogens, and they physically block pathogens from entering the body. The skin is the most important mechanical barrier. In fact, it is the single most important defense the body has. The outer layer of skin, the epidermis, is tough and very difficult for pathogens to penetrate. It consists of dead cells that are constantly being shed from the body surface. This helps remove bacteria and other infectious agents that have adhered to the skin. The epidermis also lacks blood vessels and is usually lacking moisture, so it does not provide a suitable environment for most pathogens. Hair, which is an accessory organ of the skin, also helps to keep out pathogens. Hairs inside the nose may trap larger pathogens and other particles in the air before they can enter the airways of the respiratory system.
Mucous membranes provide a mechanical barrier to pathogens and other particles at body openings. These membranes also line the respiratory, gastrointestinal, urinary, and reproductive tracts. Mucous membranes secrete mucus, which is a slimy and somewhat sticky substance that traps pathogens. Many mucous membranes also have hair-like cilia that sweep mucus and trapped pathogens toward body openings where they can be removed from the body. When you sneeze or cough, mucus, and pathogens are mechanically ejected from the nose and throat, as you can see in the photo below. Other mechanical defenses include tears, which wash pathogens from the eyes, and urine, which flushes pathogens out of the urinary tract.
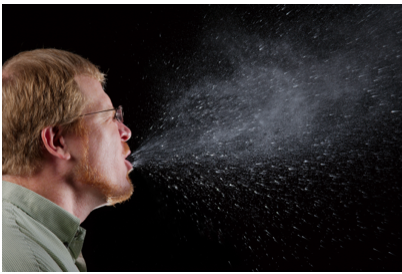
Chemical Barriers
Chemical barriers also protect against infection by pathogens. They destroy pathogens on the outer body surface, at body openings, and on inner body linings. Sweat, mucus, tears, saliva, and breastmilk all contain antimicrobial substances, such as the enzyme lysozyme, that kill pathogens, especially bacteria. Sebaceous glands in the dermis of the skin secrete acids that form a very fine, slightly acidic film on the surface of the skin that acts as a barrier to bacteria, viruses, and other potential contaminants that might penetrate the skin. Urine and vaginal secretions are also too acidic for many pathogens to endure. Semen contains zinc, which most pathogens cannot tolerate, as well as defensins, which are antimicrobial proteins that act mainly by disrupting bacterial cell membranes. In the stomach, stomach acid and digestive enzymes called proteases, which break down proteins, kill most pathogens that enter the gastrointestinal tract in food or water.
Biological Barriers
Biological barriers are living organisms that help protect the body from pathogens. Trillions of harmless bacteria normally live on the human skin and in the urinary, reproductive, and gastrointestinal tracts. These bacteria use up food and surface space that help prevent pathogenic bacteria from colonizing the body. Some of these harmless bacteria also secrete substances that change the conditions of their environment, making it less hospitable to potentially harmful bacteria. For example, they may release toxins or change the pH. All of these effects of harmless bacteria reduce the chances that pathogenic microorganisms will be able to reach sufficient numbers to cause illness.
Inflammation
If pathogens manage to breach the barriers protecting the body, then one of the first active responses of the innate immune system kicks in. This response is inflammation. The main function of inflammation is to establish a physical barrier against the spread of infection. It also eliminates the initial cause of cell injury, clears out dead cells and tissues damaged from the original insult and the inflammatory process, and initiates tissue repair. Inflammation is often a response to infection by pathogens, but there are other possible causes, including burns, frostbite, and exposure to toxins.
The signs and symptoms of inflammation include redness, swelling, warmth, pain, and frequently some loss of function. These symptoms are caused by increased blood flow into infected tissue and a number of other processes, illustrated in the figure below.
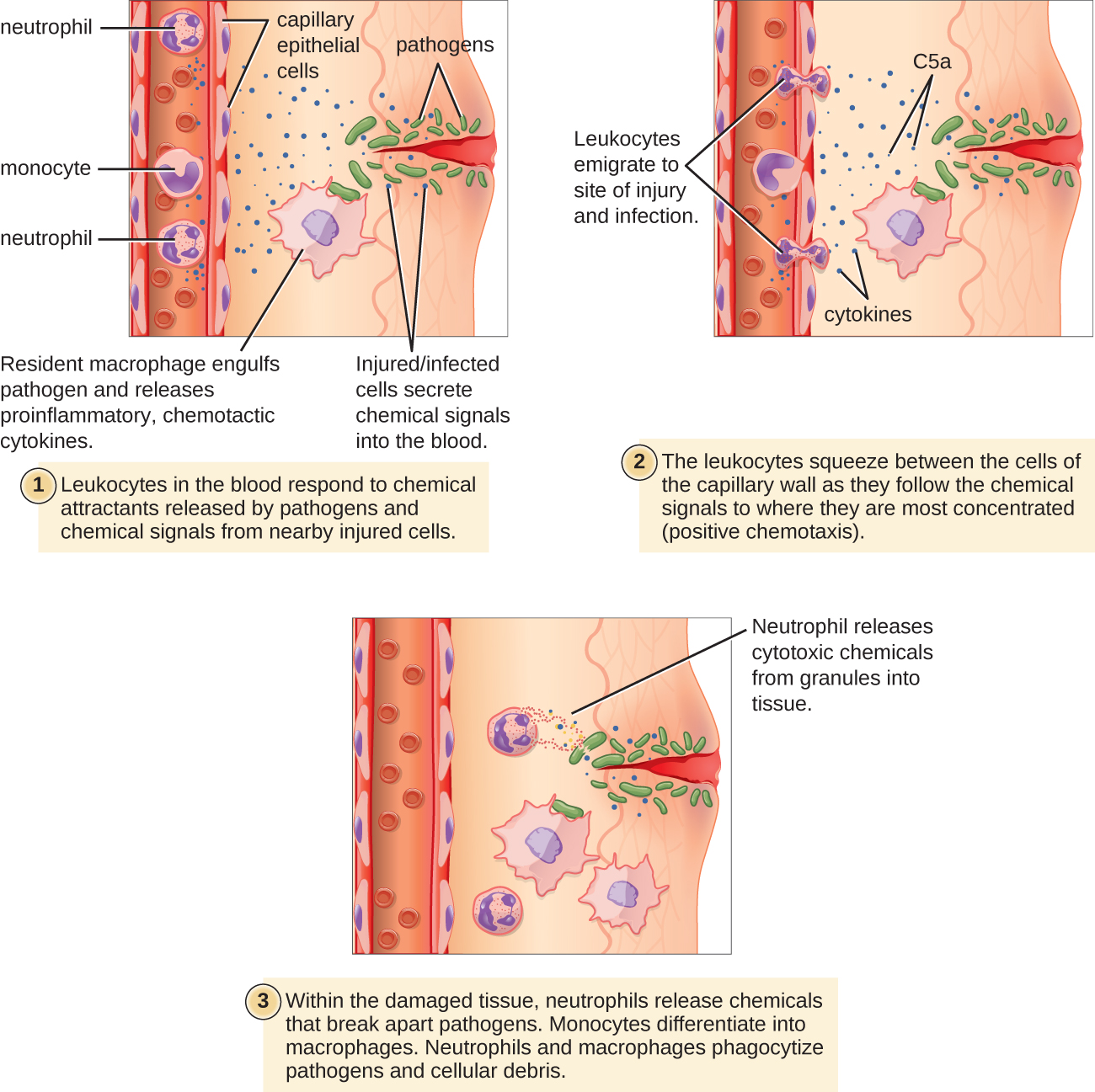
Inflammation is triggered by chemicals such as cytokines and histamines, which are released by injured or infected cells or by immune system cells such as macrophages (described in Figure 20.4.520.4.5) that are already present in tissues. These chemicals cause capillaries to dilate and become leaky, increasing blood flow to the infected area and allowing blood to enter the tissues. Pathogen-destroying leukocytes and tissue-repairing proteins migrate into tissue spaces from the bloodstream to attack pathogens and repair their damage. Cytokines also promote chemotaxis, which is migration to the site of infection by leukocytes that destroy pathogens. Some cytokines have anti-viral effects, such as shutting down protein synthesis in host cells, which viruses need in order to survive and replicate.
Complement System
The complement system is a complex biochemical mechanism named for its ability to “complement” the killing of pathogens by antibodies, which are produced as part of an adaptive immune response. The complement system consists of more than two dozen proteins that are normally found in the blood and synthesized in the liver. The proteins usually circulate as non-functional precursor molecules until activated.
As shown in Figure 20.4.420.4.4, when the first protein in the complement series is activated —typically by the binding of an antibody to an antigen on a pathogen — it sets in motion a domino effect. Each component takes its turn in a precise chain of steps known as the complement cascade. The end product is a cylinder that punctures a hole in the pathogen’s cell membrane. This allows fluids and molecules to flow in and out of the cell, which swells and bursts.

Cellular Responses
Cellular responses of the innate immune system involve a variety of different types of leukocytes. Many of these leukocytes circulate in the blood and act like independent, single-celled organisms, searching out and destroying pathogens in the human host. These and other immune cells of the innate system identify pathogens or debris and then help to eliminate them in some way. One way is by phagocytosis.
Phagocytosis
Phagocytosis is an important feature of innate immunity that is performed by cells classified as phagocytes. In the process of phagocytosis, phagocytes engulf and digest pathogens or other harmful particles. Phagocytes generally patrol the body searching for pathogens, but they can also be called to specific locations by the release of cytokines when inflammation occurs. Some phagocytes reside permanently in certain tissues.
As shown in the figure below, when a pathogen such as a bacterium is encountered by a phagocyte, the phagocyte extends a portion of its plasma membrane, wrapping the membrane around the pathogen until it is enveloped. Once inside the phagocyte, the pathogen becomes enclosed within an intracellular vesicle called a phagosome. The phagosome then fuses with another vesicle called a lysosome, forming a phagolysosome. Digestive enzymes and acids from the lysosome kill and digest the pathogen in the phagolysosome. The final step of phagocytosis is the excretion of soluble debris from the destroyed pathogen through exocytosis.
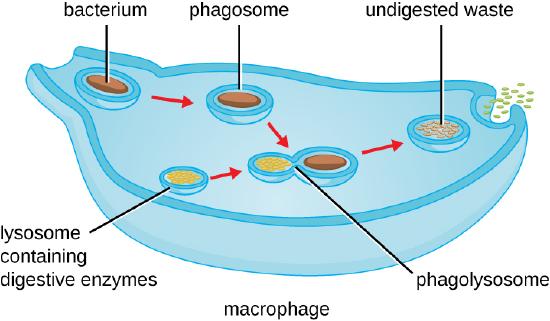
Leukocytes
Types of leukocytes that kill pathogens by phagocytosis include neutrophils, macrophages, and dendritic cells. Macrophages and dendritic cells are the derivatives of monocytes.
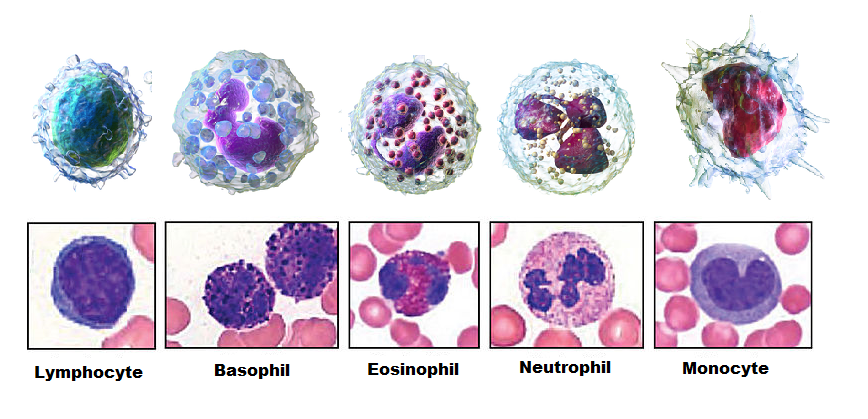
Neutrophils
Neutrophils are leukocytes that travel throughout the body in the blood and are usually the first immune cells to arrive at the site of an infection. They are the most numerous types of phagocytes and normally make up at least half of the total circulating leukocytes. The bone marrow of a normal healthy adult produces more than 100 billion neutrophils per day. During acute inflammation, more than 10 times that many neutrophils may be produced each day. Many neutrophils are needed to fight infections because after a neutrophil phagocytizes just a few pathogens, it generally dies.
Macrophages
Macrophages are large phagocytic leukocytes that develop from monocytes. Macrophages spend much of their time within the interstitial fluid in tissues of the body. They are the most efficient phagocytes and can phagocytize a substantial number of pathogens or other cells. Macrophages are also versatile cells that produce a wide array of chemicals — including enzymes, complement proteins, and cytokines — in addition to their phagocytic action. As phagocytes, macrophages act as scavengers that rid tissues of worn-out cells and other debris as well as pathogens. In addition, macrophages act as antigen-presenting cells that activate the adaptive immune system. (To learn more about antigen-presenting cells, see the concept Adaptive Immune System.)
Eosinophils
Eosinophils are non-phagocytic leukocytes that are related to neutrophils. They specialize in defending against parasites. They are very effective in killing large parasites such as worms by secreting a range of highly toxic substances when activated. Eosinophils may become overactive and cause allergies or asthma.
Basophils
Basophils are non-phagocytic leukocytes that are also related to neutrophils. They are the least numerous of all white blood cells. Basophils secrete two types of chemicals that aid in body defenses: histamines and heparin. Histamines are responsible for dilating blood vessels and increasing their permeability in inflammation. Heparin inhibits blood clotting and also promotes the movement of leukocytes into an area of infection.
Dendritic Cells
Like macrophages, dendritic cells develop from monocytes. They reside in tissues that have contact with the external environment, so they are located mainly in the skin, nose, lungs, stomach, and intestines. Besides engulfing and digesting pathogens, dendritic cells also act as antigen-presenting cells that trigger adaptive immune responses.
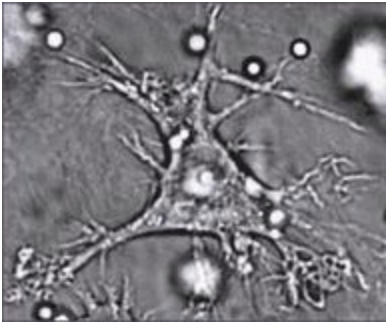
Mast Cells
Mast cells are non-phagocytic leukocytes that help to initiate inflammation by secreting histamines. In some people, histamines trigger allergic reactions as well as inflammation. Mast cells may also secrete chemicals that help defend against parasites.
Natural Killer Cells
Natural killer cells are in the subset of leukocytes called lymphocytes, which are produced by the lymphatic system. Natural killer cells destroy cancerous or virus-infected host cells, although they do not directly attack invading pathogens. Natural killer cells recognize these host cells by a condition they exhibit called “missing self.” Cells with missing self have abnormally low levels of cell-surface proteins of the major histocompatibility complex (MHC), which normally identify body cells as self.
Innate Immune Evasion
Many pathogens have evolved mechanisms that allow them to evade the innate immune system of human hosts. Some of these mechanisms include:
- invading host cells to replicate so they are “hidden” from the immune system. The bacterium that causes tuberculosis uses this mechanism.
- forming a protective capsule around themselves to avoid being destroyed by immune system cells. This defense occurs in bacteria such as Salmonella species.
- mimicking host cells so the immune system does not recognize them as foreign. Some species of Staphylococcus bacteria use this mechanism.
- directly killing phagocytes. This ability evolved in several species of bacteria, including the species that causes anthrax.
- producing molecules that prevent the formation of interferons, which are immune chemicals that fight viruses. Some influenza viruses have this capability.
- forming complex biofilms that provide protection from the cells and proteins of the immune system. This ability characterizes some species of bacteria and fungi. You can see an example of a bacterial biofilm on teeth in the figure below.

Adaptive Immune System
The Kiss of Death
The photomicrograph in Figure 20.5.120.5.1 shows a group of killer T cells (green and red) surrounding a cancer cell (blue, center). When a killer T cell makes contact with the cancer cell, it attaches to and spreads over the dangerous target. The killer T cell then uses special chemicals stored in vesicles (red) to deliver the killing blow. This event has thus been nicknamed “the kiss of death.” After the target cell is killed, the killer T cells move on to find the next victim. Killer T cells like these are important players in the adaptive immune system.
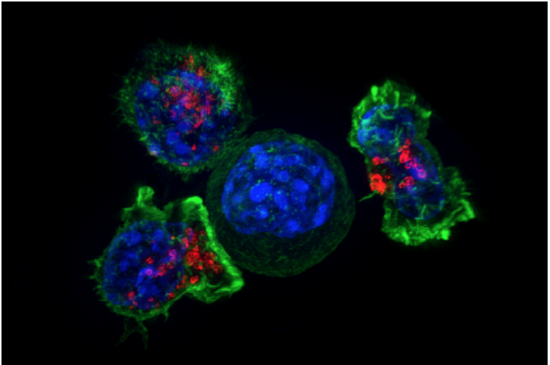
The adaptive immune system is a subsystem of the overall immune system. It is composed of highly specialized cells and processes that eliminate specific pathogens and tumor cells. An adaptive immune response is set in motion by antigens that the immune system recognizes as foreign. Unlike an innate immune response, an adaptive immune response is highly specific to a particular pathogen (or its antigen). An important function of the adaptive immune system that is not shared by the innate immune system is the creation of immunological memory or immunity. This occurs after the initial response to a specific pathogen. It allows a faster, stronger response on subsequent encounters with the same pathogen, usually before the pathogen can cause symptoms of illness.
Lymphocytes are the main cells of the adaptive immune system. They are leukocytes that arise and mature in organs of the lymphatic system, including the bone marrow and thymus. The human body normally has about 2 trillion lymphocytes, which constitute about a third of all leukocytes. Most of the lymphocytes are normally sequestered within tissue fluid or organs of the lymphatic system, including the tonsils, spleen, and lymph nodes. Only about 2 percent of the lymphocytes are normally circulating in the blood. There are two main types of lymphocytes involved in adaptive immune responses, called T cells and B cells. T cells destroy infected cells or release chemicals that regulate immune responses. B cells secrete antibodies that bind with antigens of pathogens so they can be removed by other immune cells or processes.
T Cells
There are multiple types of T cells or T lymphocytes. Major types are killer (or cytotoxic) T cells and helper T cells. Both types develop from immature T cells that become activated by exposure to an antigen.
T Cell Activation
T cells must be activated to become either killer T cells or helper T cells. This requires the presentation of a foreign antigen by antigen-presenting cells, as shown in the figure below. Antigen-presenting cells may be dendritic cells, macrophages, or B cells. Activation occurs when T cells are presented with a foreign antigen coupled with an MHC self-antigen. Helper T cells are more easily activated than killer T cells. Activation of killer T cells is strongly regulated and may require additional stimulation from helper T cells.
Figure 20.5.220.5.2: Exposure to a foreign antigen on an antigen-presenting cell is necessary to activate T cells to become killer T cells or helper T cells.
Killer T Cells (Cytotoxic T Cells)
Activated killer T cells induce the death of cells that bear a specific non-self antigen because they are infected with pathogens or are cancerous. The antigen targets the cell for destruction by killer T cells, which travel through the bloodstream searching for target cells to kill. Killer T cells may use various mechanisms to kill target cells. One way is by releasing toxins in granules that enter and kill infected or cancerous cells (Figure 20.5.320.5.3).

Helper T Cells
Activated helper T cells do not kill infected or cancerous cells. Instead, their role is to “manage” both innate and adaptive immune responses by directing other cells to perform these tasks. They control other cells by releasing cytokines. These are proteins that can influence the activity of many cell types, including killer T cells, B cells, and macrophages. For example, some cytokines released by helper T cells help activate killer T cells
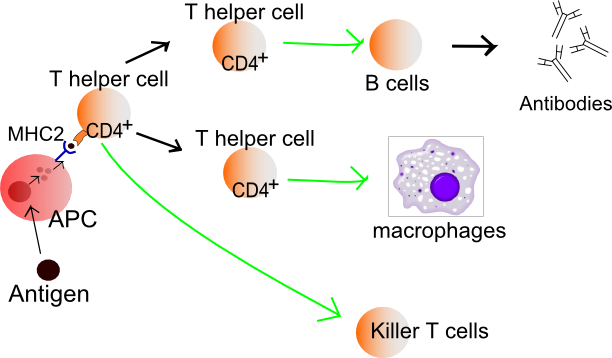
B Cells and B Cell Activation
B cells, or B lymphocytes, are the major cells involved in the creation of antibodies that circulate in blood plasma and lymph. Antibodies are large, Y-shaped proteins used by the immune system to identify and neutralize foreign invaders. Besides producing antibodies, B cells may also function as antigen-presenting cells or secrete cytokines that help control other immune cells and responses.
Before B cells can actively function to defend the host, they must be activated. As shown in Figure 20.5.520.5.5, B cell activation begins when a B cell engulfs and digests an antigen. The antigen may be either free-floating in the lymph or it may be presented by an antigen-presenting cell such as a dendritic cell or macrophage. In either case, the B cell then displays antigen fragments bound to its own MHC antigens. The MHC-antigen complex on the B cell attracts helper T cells. The helper T cells, in turn, secrete cytokines that help the B cell to multiply and the daughter cells to mature into plasma cells.
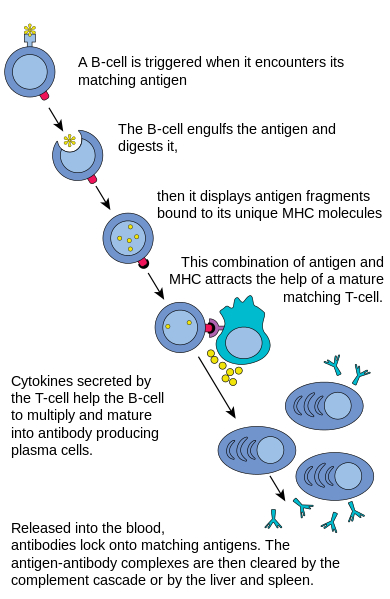
Plasma Cells
Plasma cells are antibody-secreting cells that form from activated B cells. Each plasma cell is like a tiny antibody factory. It may secrete millions of copies of an antibody, each of which can bind to the specific antigen that activated the original B cell. The specificity of an antibody to a specific antigen is illustrated in the figure below. When antibodies bind with antigens, it makes the cells bearing them easier targets for phagocytes to find and destroy. Antibody-antigen complexes may also trigger the complement system of the innate immune system, which destroys the cells in a cascade of protein enzymes. In addition, the complexes are likely to clump together (agglutinate). If this occurs, they are filtered out of the blood in the spleen or liver.
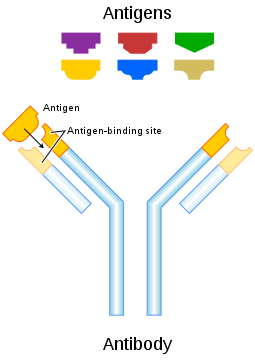
Immunity
Most activated T cells and B cells die within a few days once a pathogen has been cleared from the body. However, a few of the cells survive and remain in the body as memory T cells or memory B cells. These memory cells are ready to activate an immediate response if they are exposed to the same antigen again in the future. This is the basis of immunity.
The earliest known reference to the concept of immunity relates to the bubonic plague (see Figure 20.5.720.5.7). In 430 B.C., a Greek historian and general named Thucydides noted that people who had recovered from a previous bout of the plague could nurse people sick with the plague without contracting the illness a second time. We now know that this is true of many diseases and it occurs because of active immunity.
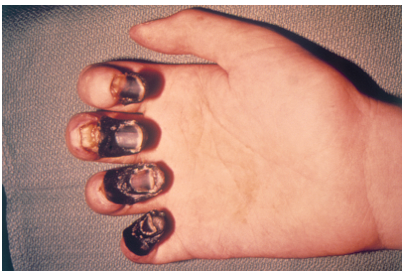
Active Immunity
Active immunity is the ability of the adaptive immune system to resist a specific pathogen because it has formed an immunological memory of the pathogen. Active immunity is adaptive because it occurs during the lifetime of an individual as an adaptation to infection with a specific pathogen and prepares the immune system for future challenges from that pathogen. Active immunity can come about naturally or artificially.
Naturally Acquired Active Immunity
Active immunity is acquired naturally when a pathogen invades the body and activates the adaptive immune system. When the initial infection is over, memory B cells and memory T cells remain that provide immunological memory of the pathogen. As long as the memory cells are alive, the immune system is ready to mount an immediate response if the same pathogen tries to infect the body again.
Artificially Acquired Active Immunity
Active immunity can also be acquired artificially through immunization. Immunization is the deliberate exposure of a person to a pathogen in order to provoke an adaptive immune response and the formation of memory cells specific to that pathogen. The pathogen is introduced in a vaccine — usually by injection, sometimes by nose or mouth (see the photo below) — so immunization is also called vaccination.

In a vaccine, only part of a pathogen, a weakened form of the pathogen, or a dead pathogen is typically used. This causes an adaptive immune response without making the immunized person sick. This is how you most likely became immune to diseases such as measles, mumps, and chickenpox. Immunizations may last for a lifetime or require periodic booster shots to maintain immunity. While immunization generally has long-lasting effects, it usually takes several weeks to develop full immunity.
Immunization is the most effective method ever discovered in preventing infectious diseases. As many as 3 million deaths are prevented each year because of vaccinations. Widespread immunity due to vaccinations is largely responsible for the worldwide eradication of smallpox and the near elimination of several other infectious diseases from many populations, including such diseases as polio and measles. Immunization is so successful because it exploits the natural specificity and inducibility of the adaptive immune system.
Passive Immunity
Passive immunity results when pathogen-specific antibodies or activated T cells are transferred to a person who has never been exposed to the pathogen. Passive immunity provides immediate protection from a pathogen, but the adaptive immune system does not develop immunological memory to protect the host from the same pathogen in the future. Unlike active immunity, passive immunity lasts only as long as the transferred antibodies or T cells survive in the blood. This is usually between a few days and a few months. However, like active immunity, passive immunity can be acquired both naturally and artificially.
Naturally Acquired Passive Immunity
Passive immunity is acquired naturally by a fetus through its mother’s blood. Antibodies are transported from mother to fetus across the placenta, so babies have high levels of antibodies at birth. Their antibodies have the same range of antigen specificity as their mother’s. Passive immunity may also be acquired by an infant through the mother’s breast milk. This gives young infants protection from common pathogens in their environment while their own immune system matures.
Artificially Acquired Passive Immunity
Older children and adults can acquire passive immunity artificially through the injection of antibodies or activated T cells. This may be done when there is a high risk of infection and insufficient time for the body to develop active immunity through vaccination. It may also be done to reduce symptoms of ongoing disease or to compensate for immunodeficiency diseases (for the latter, see the concept Disorders of the Immune System).
Adaptive Immune Evasion
Many pathogens have been around for a long time, living with human populations for generations. To persist, some have evolved mechanisms to evade the adaptive immune system of human hosts. One way they have done this is by rapidly changing their non-essential antigens. This is called antigenic variation. An example of a pathogen that takes this approach is the human immunodeficiency virus (HIV). It mutates rapidly so the proteins on its viral envelope are constantly changing. By the time the adaptive immune system responds, the virus’s antigens have changed. Antigenic variation is the main reason that efforts to develop a vaccine against HIV have not yet been successful.
Another evasion approach some pathogens may take is to mask pathogen antigens with host molecules so the host’s immune system cannot detect the antigens. HIV takes this approach as well. The envelope that covers the virus is formed from the outermost membrane of the host cell.
Feature: My Human Body
If you think that immunizations are just for kids, think again. There are several vaccines recommended by the CDC for people over the age of 18. This link shows the vaccine schedule recommended for all adults aged 19 years and older. Additional vaccines may be recommended for certain adults based on specific medical conditions or other indications. Are you up to date with your vaccines? You can check with your doctor to be sure.
Disorders of the Immune System
Allergies
An allergy is a disorder in which the immune system makes an inflammatory response to a harmless antigen. It occurs when the immune system is hypersensitive to an antigen in the environment that causes little or no response in most people. Allergies are strongly familial: allergic parents are more likely to have allergic children and those children’s allergies are likely to be more severe. This is evidence that there is a heritable tendency to develop allergies. Allergies are more common in children than adults because many children outgrow their allergies by adulthood.
Allergens
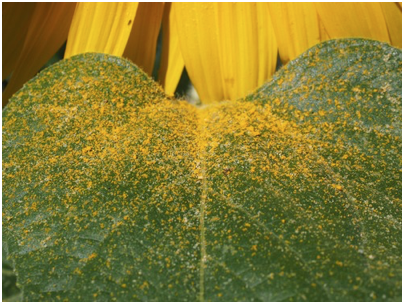
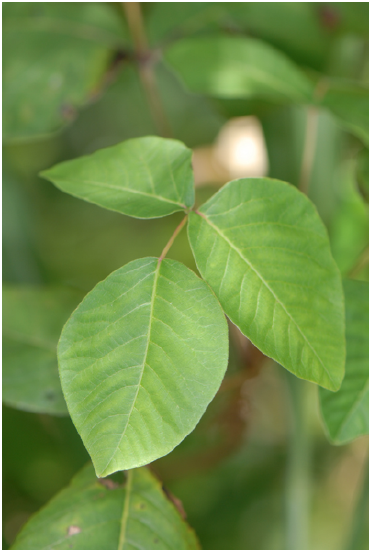
Any antigen that causes an allergy is called an allergen. Common allergens are plant pollens, dust mites, mold, specific foods (such as peanuts or shellfish), insect stings, and certain common medications (such as aspirin and penicillin). Allergens may be inhaled or ingested, or they may come into contact with the skin or eyes. Symptoms vary depending on the type of exposure and the severity of the immune system response. Two common causes of allergies are ragweed and poison ivy. Inhaling ragweed pollen may cause symptoms of allergic rhinitis, such as sneezing and red itchy eyes. Skin contact with oils in poison ivy may cause an itchy rash. This type of allergy is called contact dermatitis.
Prevalence of Allergies
There has been a significant increase in the prevalence of allergies over the past several decades, especially in the rich nations of the world, where allergies are now very common disorders. In the developed countries, about 20 percent of people have or have had hay fever, another 20 percent have had contact dermatitis, and about 6 percent have food allergies. In the poorer nations of the world, on the other hand, allergies of all types are much less common.
One explanation for the rise in allergies in the developed world is called the hygiene hypothesis. According to this hypothesis, people in developed countries live in relatively sterile environments because of hygienic practices and sanitation systems. As a result, people in these countries are exposed to fewer pathogens than their immune system evolved to cope with. To compensate, their immune system “keeps busy” by attacking harmless antigens in allergic responses.
How Allergies Occur
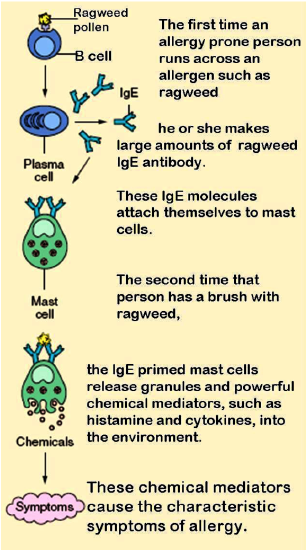
The diagram in Figure 20.6.320.6.3 shows how an allergic reaction occurs. At the first exposure to an allergen, B cells are activated to form plasma cells that produce large amounts of antibodies to the allergen. These antibodies attach to leukocytes called mast cells. Subsequently, every time the person encounters the allergen again, the mast cells are already primed and ready to deal with it. The primed mast cells immediately release cytokines and histamines, which in turn cause inflammation and recruitment of leukocytes, among other responses. These responses are responsible for the signs and symptoms of allergies.
Treating Allergies
The symptoms of allergies can range from mild to life-threatening. Mild allergy symptoms are often treated with antihistamines. These are drugs that reduce or eliminate the effects of the histamines that produce allergy symptoms.
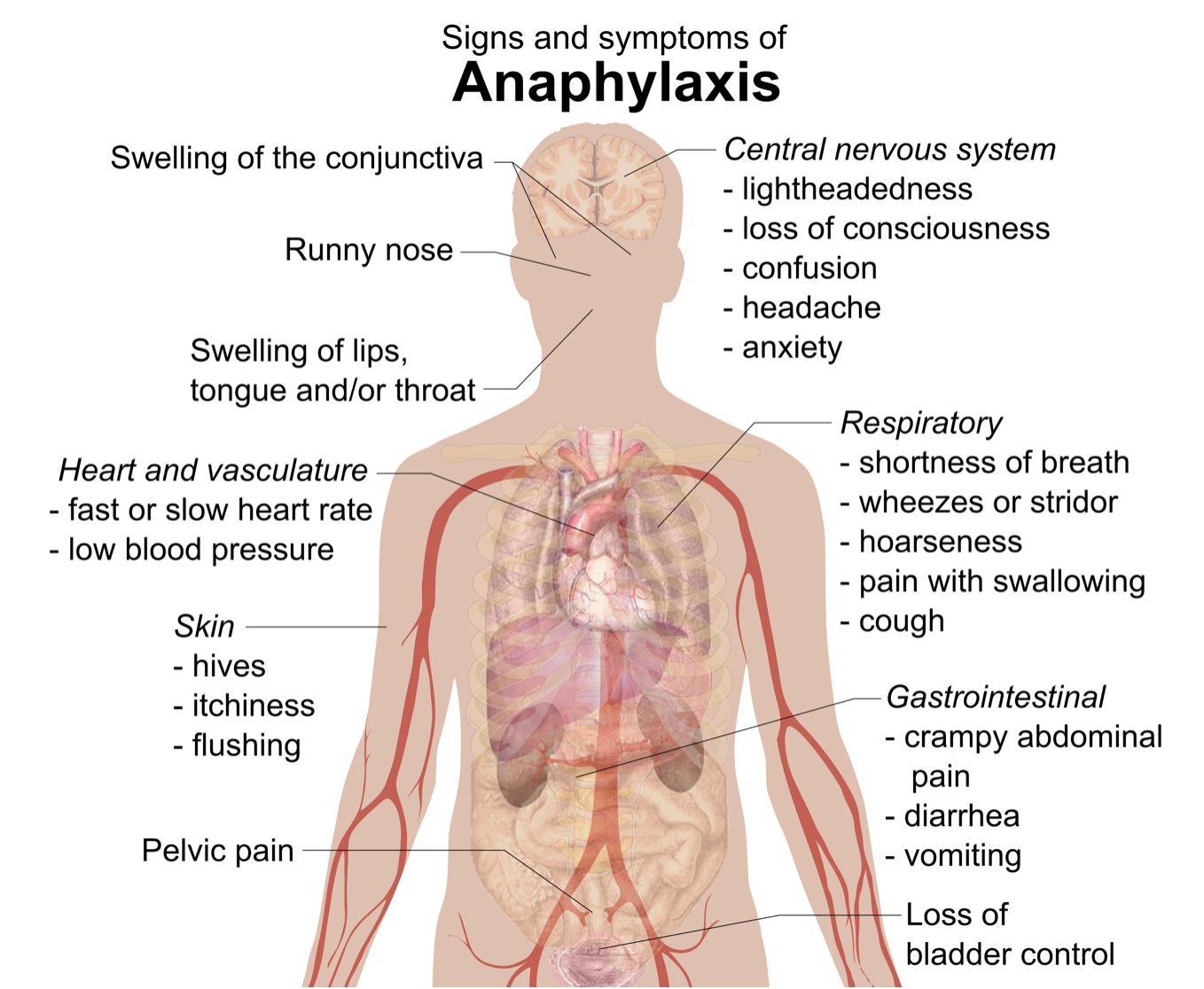
Treating Anaphylaxis
The most severe allergic reaction is a systemic reaction called anaphylaxis. This is a life-threatening response caused by a massive release of histamines. Many of the signs and symptoms of anaphylaxis are shown in the figure below. Some of them include a drop in blood pressure, changes in heart rate, shortness of breath, and swelling of the tongue and throat, which may threaten the patient with suffocation unless emergency treatment is given. People who have had anaphylactic reactions may carry an epinephrine autoinjector (widely known by its brand name EpiPen®) so they can inject themselves with epinephrine if they start to experience an anaphylactic response. The epinephrine helps to control the immune reaction until medical care can be provided. Epinephrine constricts blood vessels to increase blood pressure, relaxes smooth muscles in the lungs to reduce wheezing and improve breathing, modulates heart rate, and works to reduce swelling that may otherwise block the airways.
Immunotherapy for Allergies
Another way to treat allergies is called immunotherapy, commonly called “allergy shots.” This approach may actually cure specific allergies, at least for several years if not lifelong. It may be particularly beneficial for allergens such as pollen that are difficult or impossible to avoid. First, however, patients must be tested to identify the specific allergens that are causing their allergies. As shown in the photo below, this may involve scratching tiny amounts of common allergens into the skin and then observing whether there is a localized reaction to any of them. Each allergen is applied in a different numbered location on the skin so if there is a reaction, such as redness or swelling, the responsible allergens can be identified. Then, through periodic injections (usually weekly or monthly), patients are gradually exposed to larger and larger amounts of the allergens. Over time, generally from months to years, the immune system becomes desensitized to the allergens. This method of treating allergies is often effective for allergies to pollen or insect stings, but its usefulness for allergies to food is unclear.
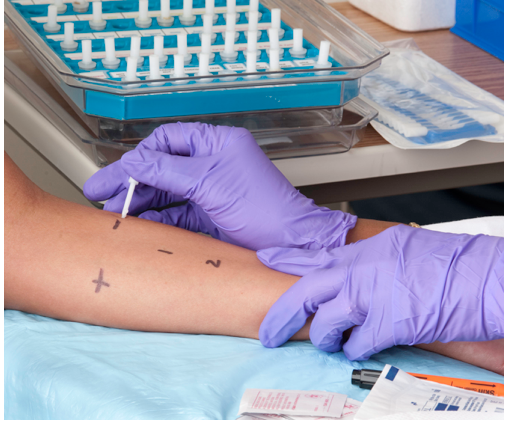
Autoimmune Diseases
Autoimmune diseases occur when the immune system fails to recognize the body’s own molecules as self. As a result, instead of ignoring the body’s healthy cells, it attacks them, causing damage to tissues and altered organ growth and function. Most often, it is B cells that are at fault in autoimmune responses. They are generally the cells that lose tolerance for self. Why does this occur? Some autoimmune diseases are thought to be caused by exposure to pathogens that have antigens similar to the body’s own molecules. After this exposure, the immune system responds to body cells as though they were pathogens as well.
Certain individuals are genetically susceptible to developing autoimmune diseases. These individuals are also more likely to develop more than one such disease. Gender is also a risk factor for autoimmunity. Females are much more likely than males to develop autoimmune diseases, probably in part because of gender differences in sex hormones.
At a population level, autoimmune diseases are less common where infectious diseases are more common. The hygiene hypothesis has been proposed to explain the inverse relationship between infectious and autoimmune diseases as well as the prevalence of allergies. According to the hypothesis, without infectious diseases to “keep it busy,” the immune system may attack the body’s own cells instead.
Common Autoimmune Diseases
An estimated 15 million or more people worldwide have one or more autoimmune diseases. Two of the most common autoimmune diseases are type I diabetes and multiple sclerosis. Both are localized diseases in terms of the specific body cells that are attacked by the immune system. In the case of type I diabetes, the immune system attacks and destroys insulin-secreting islet cells in the pancreas. In the case of multiple sclerosis, the immune system attacks and destroys the myelin sheaths that normally insulate the axons of neurons and allow rapid transmission of nerve impulses.
Some relatively common autoimmune diseases are systemic, or body-wide, diseases. They include rheumatoid arthritis and systemic lupus erythematosus (SLE). In these diseases, many tissues and organs may be attacked and injured by the immune system. For example, as you can see in the figure below, symptoms of SLE may involve the muscular, skeletal, integumentary, respiratory, and cardiovascular systems.
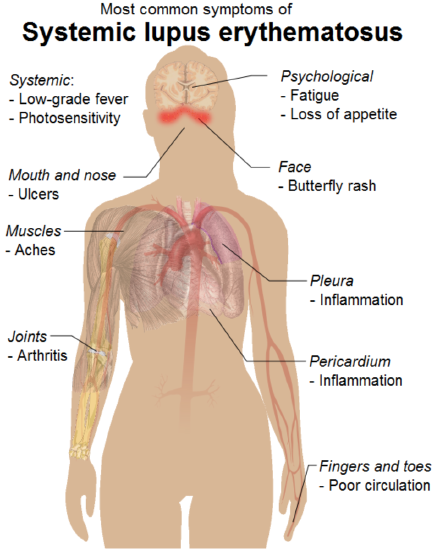
Treatment for Autoimmune Diseases
None of these common autoimmune diseases can be cured, although all of them have treatments that may help relieve symptoms and prevent some of the long-term damage they may cause. Traditional treatments for autoimmune diseases include immunosuppressive drugs to block the immune response and anti-inflammatory drugs to quell inflammation. Hormone replacement may be another option. For example, type I diabetes is treated with injections of the hormone insulin because islet cells in the pancreas can no longer secrete it.
Immunodeficiency
Immunodeficiency occurs when the immune system is not working properly, generally because one or more components of the immune system are inactive. As a result, the immune system may be unable to fight off pathogens or cancers that a normal immune system would be able to resist. Immunodeficiency may occur for a variety of reasons.
Causes of Immunodeficiency
Dozens of rare genetic diseases can result in a defective immune system. This type of immunodeficiency is called primary immunodeficiency. One is born with one of these diseases rather than acquiring it after birth. Probably the best known of these primary immunodeficiency diseases is severe combined immunodeficiency (SCID). It is also known as “bubble boy disease” because people with this disorder are extremely vulnerable to infectious diseases and some of them have become well known for living inside a bubble that provides a sterile environment. SCID is most often caused by an X-linked recessive mutation that interferes with normal B cell and T cell production.
Other types of immunodeficiency are not present at birth but acquired due to experiences or exposures that occur after birth. Acquired immunodeficiency is called secondary immunodeficiency because it is secondary to some other event or exposure. Secondary immunodeficiency may occur for a number of different reasons:
- The immune system naturally becomes less effective as people get older. This age-related decline, called immunosenescence, generally begins at about age 50 and worsens with increasing age. Immunosenescence is why older people are generally more susceptible to disease than younger people.
- The immune system may be damaged by another disorder, such as obesity, alcoholism, or the abuse of other drugs.
- In developing countries, malnutrition is the most common cause of immune system damage and immunodeficiency. Inadequate protein intake is especially damaging to the immune system. It can lead to impaired complement system activity, phagocyte malfunction, and lower-than-normal production of antibodies and cytokines.
- Surgical removal or disease of the thymus, where T lymphocytes normally mature, results in severe immunodeficiency. People without a functioning thymus are extremely susceptible to infections.
- Certain medications can suppress the immune system. This is the intended effect of immunosuppressant drugs given to people with transplanted organs so they do not reject them. In many cases, however, immunosuppression is an unwanted side effect of drugs used to treat other disorders.
- Some pathogens attack and destroy cells of the immune system. An example is a virus known as HIV, which attacks and destroys T cells.
Focus on HIV
Human immunodeficiency virus (HIV) is the most common cause of immunodeficiency in the world today, so it is the focus of the rest of this concept. It is also covered in the concept HIV and AIDS. HIV infections of human hosts are a relatively recent phenomenon. Scientists think that the virus originally infected monkeys but then jumped to human populations, probably sometime during the early to mid-1900s. This most likely occurred in West Africa, but the virus soon spread around the world. HIV was first identified by medical researchers in 1981. Since then, HIV has killed almost 40 million people worldwide, and its economic toll has also been enormous. The hardest hit countries are in Africa, where the virus has infected human populations the longest and medications to control the virus are least available.
HIV Transmission
HIV is transmitted through direct contact of mucous membranes or body fluids such as blood, semen, or breast milk. As shown in the figure below, the transmission of the virus can occur through sexual contact or the use of contaminated hypodermic needles. It can also be transmitted from an infected mother’s blood during late pregnancy or childbirth or through breast milk after birth. In the past, HIV was also transmitted occasionally through blood transfusions. Because donated blood is now screened for HIV, the virus is no longer transmitted this way.
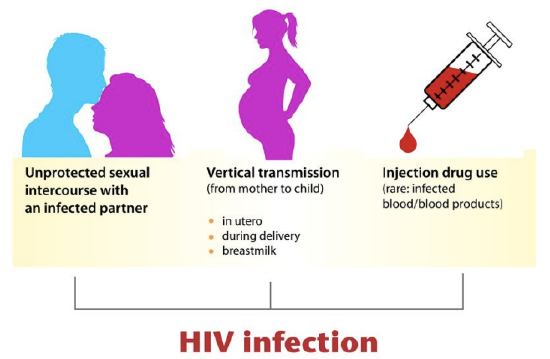
HIV and the Immune System
HIV infects and destroys helper T cells, the type of lymphocytes that regulate the immune response. How this occurs is shown in Figure 20.6.820.6.8. The virus injects its own DNA into a helper T cell and uses the T cell’s “machinery” to make copies of itself. In the process, the helper T cell is destroyed, and the virus copies go on to infect other helper T cells. HIV is able to evade the immune system and keep destroying helper T cells by mutating frequently so its surface antigens keep changing and by using the host cell’s membrane to hide its own antigens.
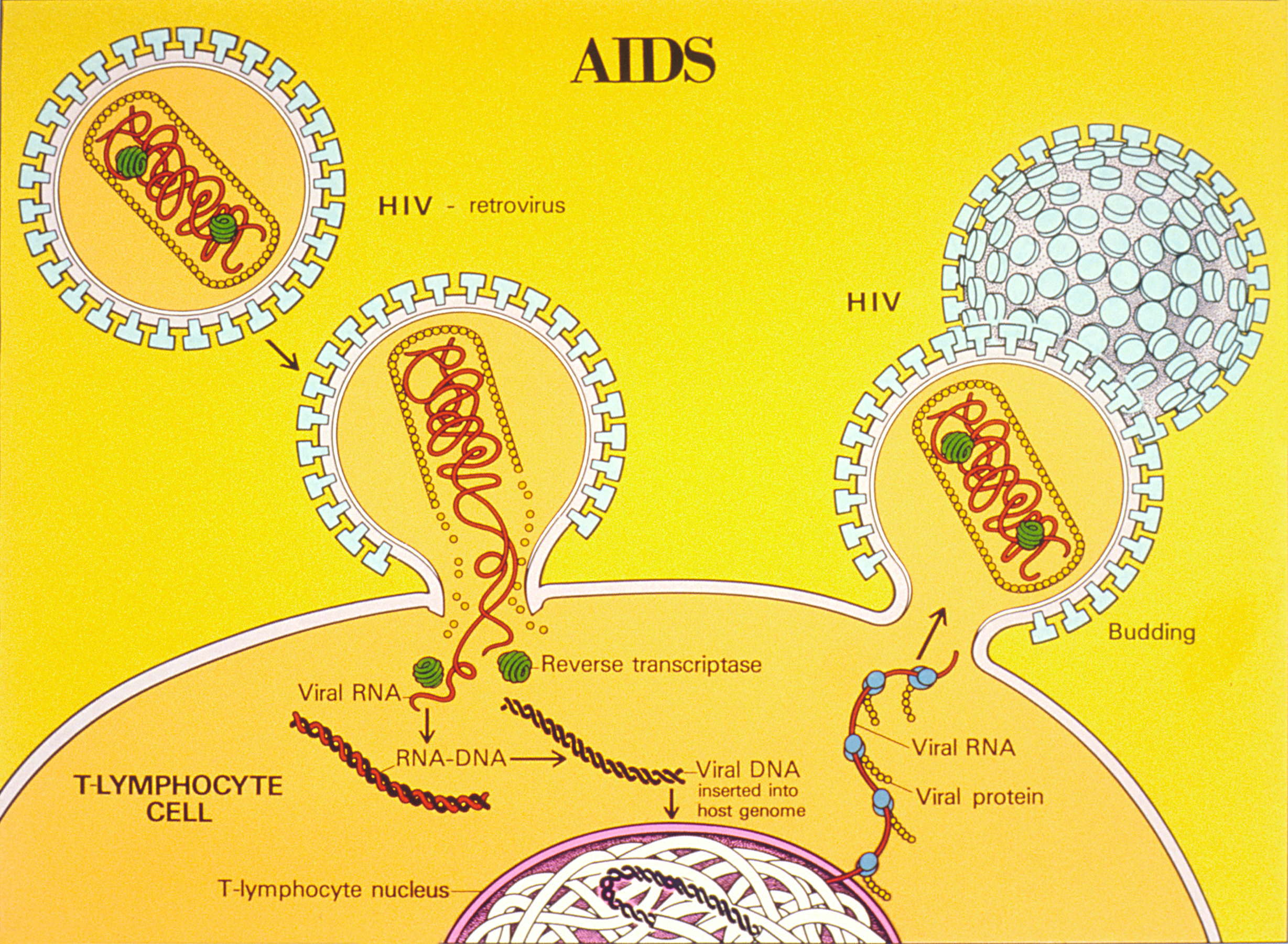
Acquired immunodeficiency syndrome (AIDS) may result from years of damage to the immune system by HIV. It occurs when helper T cells fall to a very low level and opportunistic diseases occur (see the graph below). Opportunistic diseases are infections and tumors that are rare except in people with a damaged immune system. The diseases take advantage of the “opportunity” presented by people whose immune system cannot fight back. Opportunistic diseases are usually the direct cause of death of people with AIDS.

Treating HIV/AIDS
For patients who have access to HIV medications, infection with the virus has ceased to be the death sentence that it once was. By 1995, combinations of drugs called “highly active antiretroviral therapy” were developed. For some patients, these drugs can reduce the amount of virus they are carrying to undetectable levels. However, some amount of virus always hides in the body’s immune cells and will multiply again if a patient stops taking the medications. Researchers are trying to develop drugs to kill these hidden viruses as well. If their efforts are successful, it could bring an end to AIDS.
watch
Check out this video to learn more about the immune system: