Describe the muscular, skeletal, and integumentary systems
This set of body systems has been grouped together as the “support systems.” Remember, this isn’t a hard-and-fast categorization: these systems are grouped together to help you organize your learning. These support systems provide the structure (and support!) for your body: your muscles, skeleton, and skin.
Learning Objectives
- Identify the structure and function of the muscular system
- Identify the structure and function of the skeletal system
- Identify the structure and function of the integumentary system
Muscular System
The muscular system is the biological system of humans that produces movement. The muscular system, in vertebrates, is controlled through the nervous system, although some muscles, like cardiac muscle, can be completely autonomous. Muscle is contractile tissue and is derived from the mesodermal layer of embryonic germ cells. Its function is to produce force and cause motion, either locomotion or movement within internal organs. Much of muscle contraction occurs without conscious thought and is necessary for survival, like the contraction of the heart or peristalsis, which pushes food through the digestive system. Voluntary muscle contraction is used to move the body and can be finely controlled, such as movements of the finger or gross movements like those of the biceps and triceps.
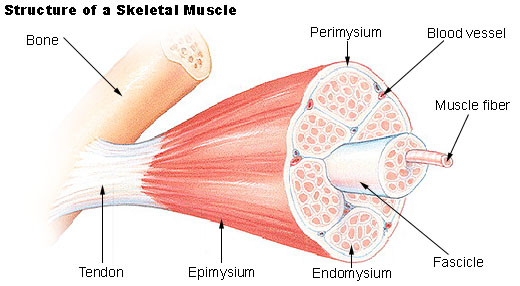
Figure 1. Muscle structure
Muscle is composed of muscle cells (sometimes known as “muscle fibers”). Within the cells are myofibrils; myofibrils contain sarcomeres which are composed of actin and myosin. Individual muscle cells are lined with endomysium. Muscle cells are bound together by perimysium into bundles called fascicles. These bundles are then grouped together to form muscle, and is lined by epimysium. Muscle spindles are distributed throughout the muscles, and provide sensory feedback information to the central nervous system.
Skeletal muscle, which involves muscles from the skeletal tissue, is arranged in discrete groups (Figure 1). An example is the biceps brachii. It is connected by tendons to processes of the skeleton. In contrast, smooth muscle occurs at various scales in almost every organ, from the skin (in which it controls erection of body hair) to the blood vessels and digestive tract (in which it controls the caliber of a lumen and peristalsis, respectively).
There are approximately 640 skeletal muscles in the human body. Contrary to popular belief, the number of muscle fibers cannot be increased through exercise; instead the muscle cells simply get bigger. It is however believed that myofibrils have a limited capacity for growth through hypertrophy and will split if subject to increased demand. There are three basic types of muscles in the body: smooth, cardiac, and skeletal (see Figure 2). While they differ in many regards, they all use actin sliding against myosin to create muscle contraction and relaxation. In skeletal muscle, contraction is stimulated at each cell by nervous impulses that releases acetylcholine at the neuromuscular junction, creating action potentials along the cell membrane. All skeletal muscle and many smooth muscle contractions are stimulated by the binding of the neurotransmitter acetylcholine. Muscular activity accounts for most of the body’s energy consumption. Muscles store energy for their own use in the form of glycogen, which represents about 1% of their mass. Glycogen can be rapidly converted to glucose when more energy is necessary.
Types

Figure 2. Smooth muscle cells do not have striations, while skeletal muscle cells do. Cardiac muscle cells have striations, but, unlike the multinucleate skeletal cells, they have only one nucleus. Cardiac muscle tissue also has intercalated discs, specialized regions running along the plasma membrane that join adjacent cardiac muscle cells and assist in passing an electrical impulse from cell to cell.
- Smooth muscle or “involuntary muscle” consists of spindle shaped muscle cells found within the walls of organs and structures such as the esophagus, stomach, intestines, bronchi, uterus, ureters, bladder, and blood vessels. Smooth muscle cells contain only one nucleus and no striations.
- Cardiac muscle is also an “involuntary muscle” but it is striated in structure and appearance. Like smooth muscle, cardiac muscle cells contain only one nucleus. Cardiac muscle is found only within the heart.
- Skeletal muscle or “voluntary muscle” is anchored by tendons to the bone and is used to effect skeletal movement such as locomotion. Skeletal muscle cells are multinucleated with the nuclei peripherally located. Skeletal muscle is called ‘striated’ because of the longitudinally striped appearance under light microscopy. Functions of the skeletal muscle include:
- Support of the body
- Aids in bone movement
- Helps maintain a constant temperature throughout the body
- Assists with the movement of cardiovascular and lymphatic vessels through contractions
- Protection of internal organs and contributing to joint stability
Cardiac and skeletal muscle are striated in that they contain sarcomeres and are packed into highly-regular arrangements of bundles; smooth muscle has neither. Striated muscle is often used in short, intense bursts, whereas smooth muscle sustains longer or even near-permanent contractions.
Skeletal muscle is further divided into several subtypes:
- Type I, slow oxidative, slow twitch, or “red” muscle is dense with capillaries and is rich in mitochondria and myoglobin, giving the muscle tissue its characteristic red color. It can carry more oxygen and sustain aerobic activity.
- Type II, fast twitch, muscle has three major kinds that are, in order of increasing contractile speed:
- Type IIa, which, like slow muscle, is aerobic, rich in mitochondria and capillaries and appears red.
- Type IIx (also known as type IId), which is less dense in mitochondria and myoglobin. This is the fastest muscle type in humans. It can contract more quickly and with a greater amount of force than oxidative muscle, but can sustain only short, anaerobic bursts of activity before muscle contraction becomes painful (often attributed to a build-up of lactic acid). N.B. in some books and articles this muscle in humans was, confusingly, called type IIB
- Type IIb, which is anaerobic, glycolytic, “white” muscle that is even less dense in mitochondria and myoglobin. In small animals like rodents or rabbits this is the major fast muscle type, explaining the pale color of their meat.
For most skeletal muscles, contraction occurs as a result of conscious effort originating in the brain. The brain sends signals, in the form of action potentials, through the nervous system to the motor neuron that innervates the muscle fiber. However, some muscles (such as the heart) do not contract as a result of conscious effort. These are said to be autonomic. Also, it is not always necessary for the signals to originate from the brain. Reflexes are fast, unconscious muscular reactions that occur due to unexpected physical stimuli. The action potentials for reflexes originate in the spinal cord instead of the brain.
There are three general types of muscle contractions, matching the types of muscles: skeletal muscle contractions, heart muscle contractions, and smooth muscle contractions.
Skeletal System
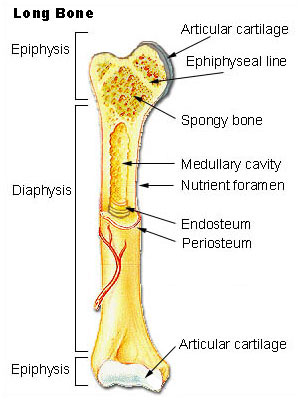
Figure 3. Parts of a long bone.
The skeletal system not only helps to provide movement and support but also serves as a storage area for calcium and inorganic salts and a source of blood cells. The adult human body has 206 bones in a variety of shapes and sizes. Basically there are 4 types of bones categorized according to shape:
- Long bones have a long longitudinal axis (Figure 3).
- Short bones have a short longitudinal axis and are more cube-like.
- Flat bones are thin and curved such as some of the bones of the skull.
- Irregular bones are often found in groups and have a variety of shapes and sizes.
Notice the long shaft or diaphysis in the middle of the bone. The diaphysis contains compact bone surrounding a medullary cavity containing bone marrow On either end is an epiphysis containing cancellous or spongy bone. The epiphyseal line is a remnant of the growth plate. The epiphyses also contain hyaline cartilage for forming joints with other bones. Surrounding the bone is a membrane called the periosteum. The periosteum contains blood vessels and cells that help to repair and restore bone.
There are also 2 types of bone tissue in different amounts in bones. Compact bone (sometimes called cortical bone) is very dense. Cancellous bone (sometimes called spongy bone) looks more like a trabeculated matrix (Figure 4). It is found in the central regions of some of the skull bones or at ends (epiphyses) of long bones. The bone forming cells (osteocytes) get their nutrients by diffusion.
Notice the spongy appearance of the trabeculated bone. The cortical bone is located near the margins of the bone and is more dense.
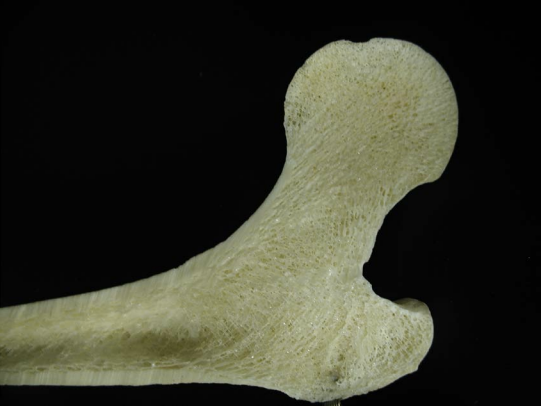
Figure 4.Trabecular and cortical bone of the femur. (Photo by Bruce Forciea).
Bone Structure
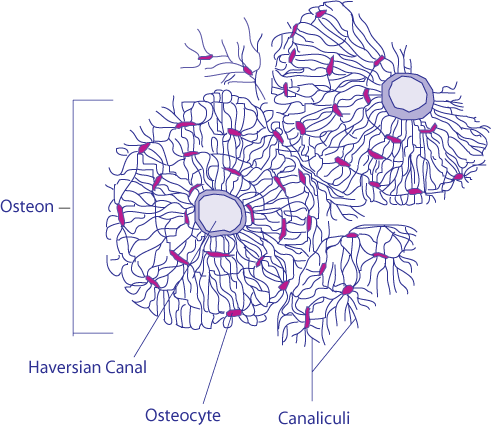
Figure 5. Haversian system.
Compact bone is organized according to structural units called Haversian systems or osteons (Figure 5). These are located along the lines of force and line up along the long axis of the bone. The Haversian systems are connected together and form an interconnected structure that provides support and strength to bones.
Haversian systems contain a central canal (Haversian canal) that serves as a pathway for blood vessels and nerves. The bone is deposited along concentric rings called lamellae. Along the lamellae are small openings called lacunae. The lacunae contain fluid and bone cells called osteocytes. Radiating out in all directions from lacunae are small canals called canaliculi. Haversian systems are interconnected by a series of larger canals called Volksmann’s canals (perforating canals).
Bone Cells
There are 3 basic types of cells in bone. Osteoblasts undergo mitosis and secrete a substance that acts as the framework for bone. Once this substance (called osteoid) is secreted minerals can deposit and form hardened bone. Osteoblasts respond to certain bone forming hormones as well as from physical stress. Osteocytes are mature osteoblasts that cannot divide by mitosis (Figure 6).
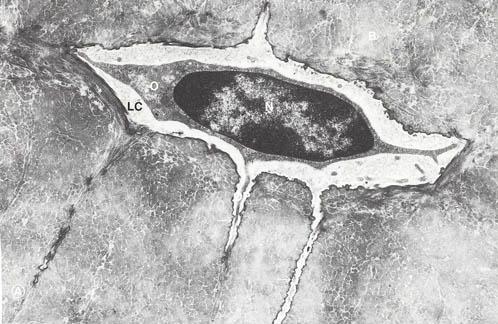
Figure 6. Osteocytes are mature osteoblasts that reside in a lacuna. They are surrounded by bony matrix.
Osteocytes reside in lacunae. Osteoclasts are capable of demineralizing bone. They free up calcium from bone to make it available to the body depending on the body’s needs.
Bone Marrow
Bone marrow is located in the medullary (marrow) cavity of long bones and in some spongy bones. There are 2 kinds of marrow. Red marrow exists in the bones of infants and children. It is called red because it contains a large number of red blood cells. In adults the red marrow is replaced by yellow marrow. It is called yellow because it contains a large proportion of fat cells. Yellow marrow decreases its ability to form new red blood cells. However, not all adult bones contain yellow marrow. The following bones continue to contain red marrow and produce red blood cells:
- Proximal end of humerus
- Ribs
- Bodies of vertebrae
- Pelvis
- Femur
The Skeleton
The skeleton is divided into 2 sections: the axial and appendicular sections (Figure 7). The axial skeleton includes the skull, spine, ribcage, and sacrum and is indicated in blue in Figure 7. The appendicular skeleton is indicated with red labels.
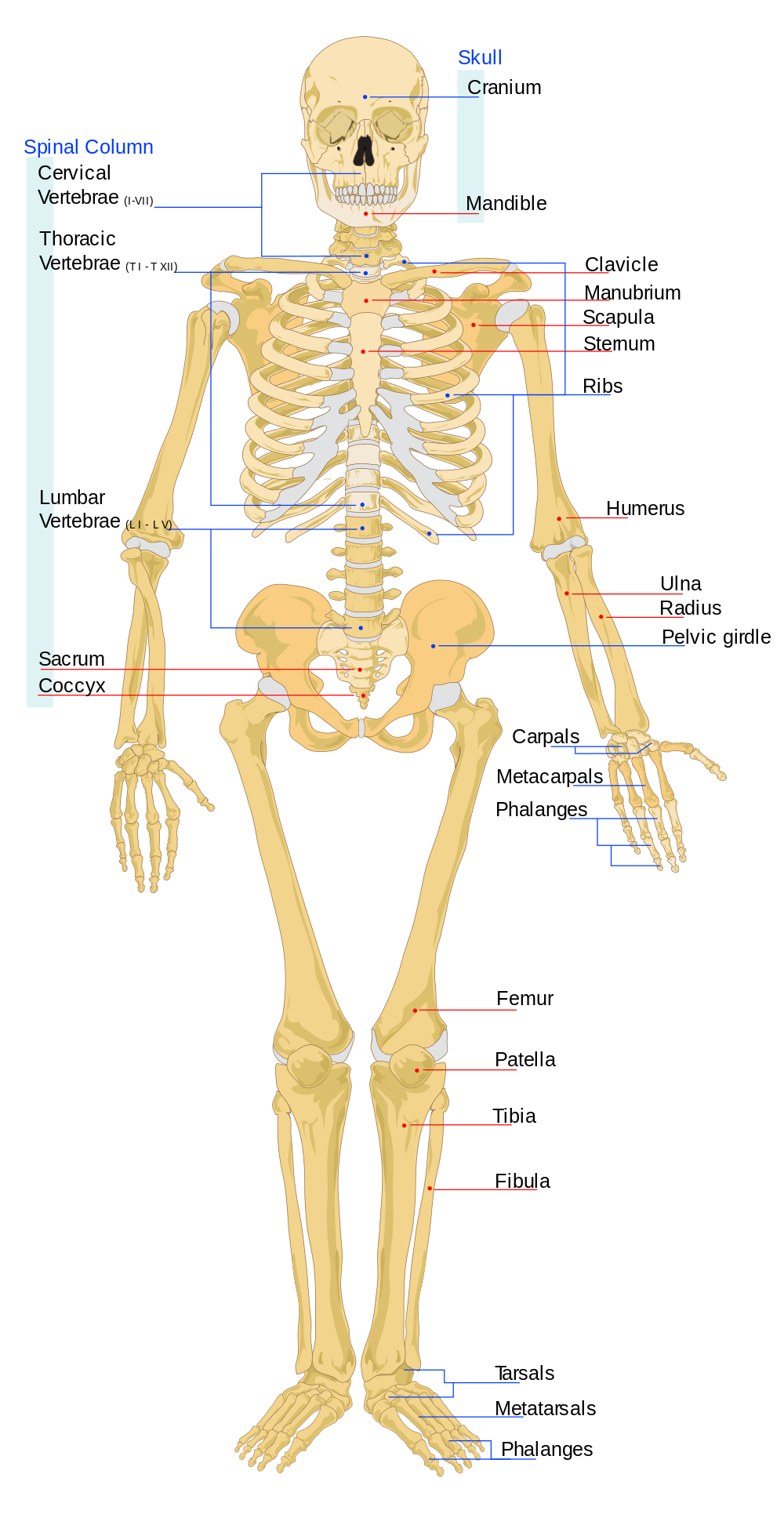
Figure 7. The skeleton.
Video Review
This video provides another introduction to the skeletal system:
Integumentary System
The integumentary system consists of the skin, hair, nails, the subcutaneous tissue below the skin, and assorted glands.The most obvious function of the integumentary system is the protection that the skin gives to underlying tissues. The skin not only keeps most harmful substances out, but also prevents the loss of fluids.
A major function of the subcutaneous tissue is to connect the skin to underlying tissues such as muscles. Hair on the scalp provides insulation from cold for the head. The hair of eyelashes and eyebrows helps keep dust and perspiration out of the eyes, and the hair in our nostrils helps keep dust out of the nasal cavities. Nails protect the tips of fingers and toes from mechanical injury. Fingernails give the fingers greater ability to pick up small objects.
There are four types of glands in the integumentary system: sudoriferous (sweat) glands, sebaceous glands, ceruminous glands, and mammary glands. These are all exocrine glands, secreting materials outside the cells and body. Sudoriferous glands are sweat producing glands. These are important to help maintain body temperature. Sebaceous glands are oil producing glands which help inhibit bacteria, keep us waterproof and prevent our hair and skin from drying out. Ceruminous glands produce earwax which keeps the outer surface of the eardrum pliable and prevents drying. Mammary glands produce milk.
Skin
In zoology and dermatology, skin is an organ of the integumentary system made up of a layer of tissues that guard underlying muscles and organs. As the interface with the surroundings, it plays the most important role in protecting against pathogens. Its other main functions are insulation and temperature regulation, sensation and vitamin D and B synthesis. Skin is considered one of the most important parts of the body.
Skin has pigmentation known as melanin that is provided by melanocytes. Melanin absorbs some of the potentially dangerous radiation in sunlight. It also contains DNA repair enzymes which reverse UV damage, and people who lack the genes for these enzymes suffer high rates of skin cancer. One form predominantly produced by UV light, malignant melanoma, is particularly invasive, causing it to spread quickly, and can often be deadly. Human skin pigmentation varies among populations in a striking manner. This has sometimes led to the classification of people(s) on the basis of skin color.
Damaged skin will try to heal by forming scar tissue, often giving rise to discoloration and depigmentation of the skin.
The skin is often known as “the largest organ in the human body.” This applies to exterior surface, as it covers the body, appearing to have the largest surface area of all the organs. Moreover, it applies to weight, as it weighs more than any single internal organ, accounting for about 15 percent of body weight. For the average adult human, the skin has a surface area of between 1.5-2.0 square meters, most of it is between 2–3 mm thick. The average square inch of skin holds 650 sweat glands, 20 blood vessels, 60,000 melanocytes, and more than a thousand nerve endings.
The use of natural or synthetic cosmetics to treat the appearance of the face and condition of the skin (such as pore control and black head cleansing) is common among many cultures.
Layers
The skin has two major layers which are made of different tissues and have very different functions.
Skin is composed of the epidermis and the dermis. Below these layers lies the hypodermis or subcutaneous adipose layer, which is not usually classified as a layer of skin.
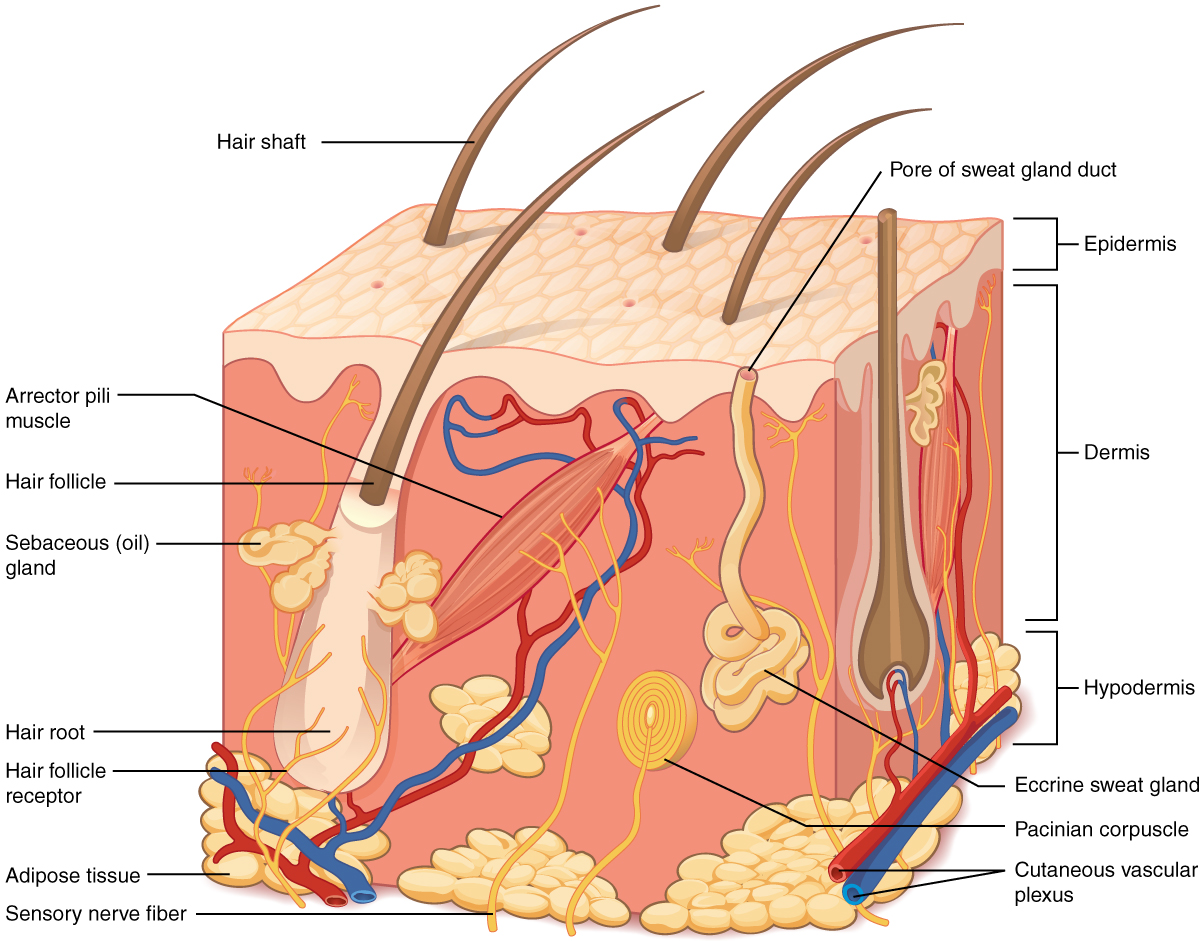
Figure 8. The skin is composed of two main layers: the epidermis, made of closely packed epithelial cells, and the dermis, made of dense, irregular connective tissue that houses blood vessels, hair follicles, sweat glands, and other structures. Beneath the dermis lies the hypodermis, which is composed mainly of loose connective and fatty tissues.
The outermost epidermis consists of stratified squamous keratinizing epithelium with an underlying basement membrane. It contains no blood vessels, and is nourished by diffusion from the dermis. The main type of cells which make up the epidermis are keratinocytes, with melanocytes and Langerhans cells also present. The epidermis can be further subdivided into the following strata (beginning with the outermost layer): corneum, lucidum, granulosum, spinosum, basale. Cells are formed through mitosis at the innermost layers. They move up the strata changing shape and composition as they differentiate, inducing expression of new types of keratin genes. They eventually reach the corneum and become sloughed off (desquamation). This process is called keratinization and takes place within about 30 days. This layer of skin is responsible for keeping water in the body and keeping other harmful chemicals and pathogens out.
Blood capillaries are found beneath the dermis, and are linked to an arteriole and a venule. Arterial shunt vessels may bypass the network in ears, the nose and fingertips.
The dermis lies below the epidermis and contains a number of structures including blood vessels, nerves, hair follicles, smooth muscle, glands and lymphatic tissue. It consists of loose connective tissue otherwise called areolar connective tissue—collagen, elastin and reticular fibers are present. Erector muscles, attached between the hair papilla and epidermis, can contract, resulting in the hair fiber pulled upright and consequentially goose bumps. The main cell types are fibroblasts, adipocytes (fat storage) and macrophages. Sebaceous glands are exocrine glands which produce, a mixture of lipids and waxy substances: lubrication, water-proofing, softening and antibactericidal actions are among the many functions of sebum. Sweat glands open up via a duct onto the skin by a pore.
The dermis is made of an irregular type of fibrous connective tissue consisting of collagen and elastin fibers. It can be split into the papillary and reticular layers. The papillary layer is outermost and extends into the epidermis to supply it with vessels. It is composed of loosely arranged fibers. Papillary ridges make up the lines of the hands giving us fingerprints. The reticular layer is more dense and is continuous with the hypodermis. It contains the bulk of the structures (such as sweat glands). The reticular layer is composed of irregularly arranged fibers and resists stretching.
The hypodermis is not part of the skin, and lies below the dermis. Its purpose is to attach the skin to underlying bone and muscle as well as supplying it with blood vessels and nerves. It consists of loose connective tissue and elastin. The main cell types are fibroblasts, macrophages and adipocytes (the hypodermis contains 95% of body fat). Fat serves as padding and insulation for the body.
Functions
- Protection: Skin gives an anatomical barrier between the internal and external environment in bodily defense; Langerhans cells in the skin are part of the immune system
- Sensation: Skin contains a variety of nerve endings that react to heat, cold, touch, pressure, vibration, and tissue injury
- Heat regulation: The skin contains a blood supply far greater than its requirements which allows precise control of energy loss by radiation, convection and conduction. Dilated blood vessels increase perfusion and heat loss while constricted vessels greatly reduce cutaneous blood flow and conserve heat. Erector pili muscles are significant in animals.
Hair
Humans have three different types of hair:
- Lanugo, the fine, unpigmented hair that covers nearly the entire body of a fetus, although most has been replaced with vellus by the time of the baby’s birth
- Vellus hair, the short, downy, “peach fuzz” body hair (also unpigmented) that grows in most places on the human body. While it occurs in both sexes, and makes up much of the hair in children, men have a much smaller percentage (around 10%) vellus whereas 2/3 of a female’s hair is vellus.
- Terminal hair, the fully developed hair, which is generally longer, coarser, thicker, and darker than vellus hair, and often is found in regions such as the axillary, male beard, and pubic.
Nails
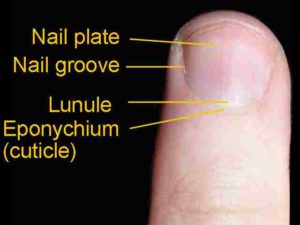
Figure 9. The parts of a finger nail
The fingernail is an important structure made of keratin. The fingernail generally serve two purposes. It serves as a protective plate and enhances sensation of the fingertip. The protection function of the fingernail is commonly known, but the sensation function is equally important. The fingertip has many nerve endings in it allowing us to receive volumes of information about objects we touch. The nail acts as a counterforce to the fingertip providing even more sensory input when an object is touched.
Nail Structure
The structure we know of as the nail is divided into six specific parts: the root, nail bed, nail plate, eponychium (cuticle), perionychium, and hyponychium.
Root The root of the fingernail is also known as the germinal matrix. This portion of the nail is actually beneath the skin behind the fingernail and extends several millimeters into the finger. The fingernail root produces most of the volume of the nail and the nail bed. This portion of the nail does not have any melanocytes, or melanin producing cells. The edge of the germinal matrix is seen as a white, crescent shaped structure called the lunula.
Nail Bed The nail bed is part of the nail matrix called the sterile matrix. It extends from the edge of the germinal matrix, or lunula, to the hyponychium. The nail bed contains the blood vessels, nerves, and melanocytes, or melanin-producing cells. As the nail is produced by the root, it streams down along the nail bed, which adds material to the undersurface of the nail making it thicker. It is important for normal nail growth that the nail bed be smooth. If it is not, the nail may split or develop grooves that can be cosmetically unappealing.
Nail Plate The nail plate is the actual fingernail, made of translucent keratin. The pink appearance of the nail comes from the blood vessels underneath the nail. The underneath surface of the nail plate has grooves along the length of the nail that help anchor it to the nail bed.
Eponychium The cuticle of the fingernail is also called the eponychium. The cuticle is situated between the skin of the finger and the nail plate fusing these structures together and providing a waterproof barrier.
Perionychium The perioncyhium is the skin that overlies the nail plate on its sides. It is also known as the paronychial edge. The perionychium is the site of hangnails, ingrown nails, and an infection of the skin called paronychia.
Hyponychium The hyponychium is the area between the nail plate and the fingertip. It is the junction between the free edge of the nail and the skin of the fingertip, also providing a waterproof barrier.
Glands
Sweat Glands
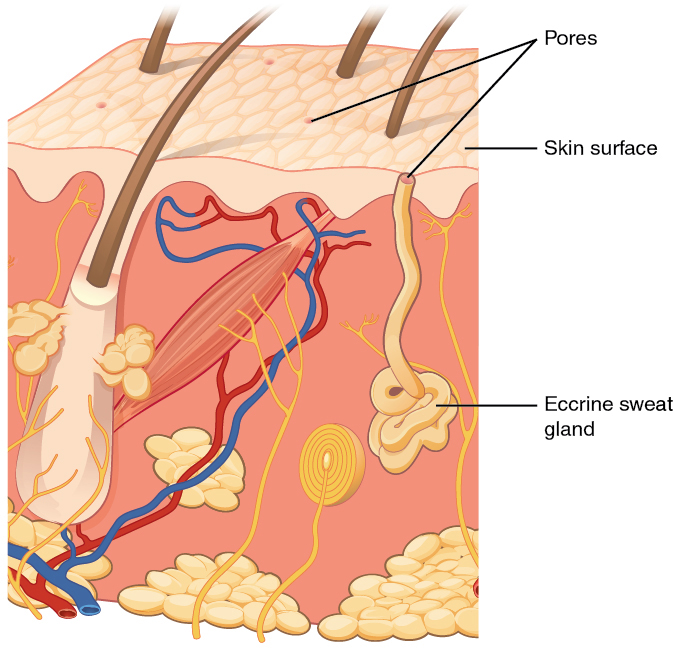
Figure 10. Eccrine glands are coiled glands in the dermis that release sweat that is mostly water.
In humans, there are two kinds of sweat glands which differ greatly in both the composition of the sweat and its purpose.
Eccrine (a.k.a. merocrine)
Eccrine sweat glands are exocrine glands distributed over the entire body surface but are particularly abundant on the palms of hands, soles of feet, and on the forehead. These produce sweat that is composed chiefly of water (99%) with various salts. The primary function is body temperature regulation.
Eccrine sweat glands are coiled tubular glands derived leading directly to the most superficial layer of the epidermis (out layer of skin) but extending into the inner layer of the skin (dermis layer). They are distributed over almost the entire surface of the body in humans and many other species, but are lacking in some marine and fur-bearing species. The sweat glands are controlled by sympathetic cholinergic nerves which are controlled by a center in the hypothalamus. The hypothalamus senses core temperature directly, and also has input from temperature receptors in the skin and modifies the sweat output, along with other thermoregulatory processes.
Human eccrine sweat is composed chiefly of water with various salts and organic compounds in solution. It contains minute amounts of fatty materials, urea, and other wastes. The concentration of sodium varies from 35–65 mmol/l and is lower in people acclimatised to a hot environment. The sweat of other species generally differ in composition.
Apocrine
Apocrine sweat glands only develop during early- to mid-puberty (approximately age 15) and release more than normal amounts of sweat for approximately a month and subsequently regulate and release normal amounts of sweat after a certain period of time. Apocrine sweat glands produce sweat that contains fatty materials. These glands are mainly present in the armpits and around the genital area and their activity is the main cause of sweat odor, due to the bacteria that break down the organic compounds in the sweat from these glands. Emotional stress increases the production of sweat from the apocrine glands, or more precisely: the sweat already present in the tubule is squeezed out. Apocrine sweat glands essentially serve as scent glands.
Sebaceous Glands
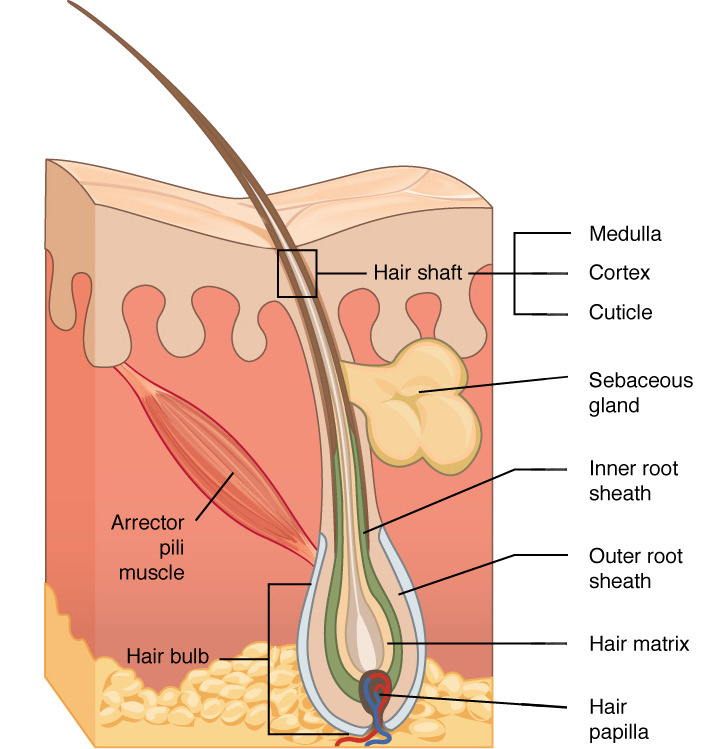
Figure 11. Hair follicles originate in the epidermis and have many different parts.
The sebaceous glands are glands found in the skin of mammals. They secrete an oily substance called sebum (Latin, meaning fat or tallow) that is made of fat (lipids) and the debris of dead fat-producing cells. These glands exist in humans throughout the skin except in the palms of the hands and soles of the feet. Sebum acts to protect and waterproof hair and skin, and keep them from becoming dry, brittle, and cracked. It can also inhibit the growth of microorganisms on skin.
Sebaceous glands can usually be found in hair-covered areas where they are connected to hair follicles to deposit sebum on the hairs, and bring it to the skin surface along the hair shaft. The structure consisting of hair, hair follicle and sebaceous gland is also known as pilosebaceous unit. Sebaceous glands are also found in non haired areas of lips, eyelids, penis, labia minora and nipples; here the sebum reaches the surface through ducts. In the glands, sebum is produced within specialized cells and is released as these cells burst; sebaceous glands are thus classified as holocrine glands.
Sebum is odorless, but its bacterial breakdown can produce odors. Sebum is the cause of some people experiencing “oily” hair if it is not washed for several days. Earwax is partly sebum, as is mucopurulent discharge, the dry substance accumulating in the corners of the eye after sleeping.
The composition of sebum varies from species to species; in humans, the lipid content consists of about 25% wax monoesters, 41% triglycerides, 16% free fatty acids, and 12% squalene.
The activity of the sebaceous glands increases during puberty because of heightened levels of androgens.
Sebaceous glands are involved in skin problems such as acne and keratosis pilaris. A blocked sebaceous gland can result in a sebaceous cyst. The prescription drug isotretinoin significantly reduces the amount of sebum produced by the sebaceous glands, and is used to treat acne. The extreme use (up to 10 times doctor prescribed amounts) of anabolic steroids by bodybuilders to prevent weight loss tend to stimulate the sebaceous glands which can cause acne.
The sebaceous glands of a human fetus in utero secrete a substance called vernix caseosa, a “waxy” or “cheesy” white substance coating the skin of newborns.
The preputial glands of mice and rats are large modified sebaceous glands that produce pheromones.
Ceruminous glands
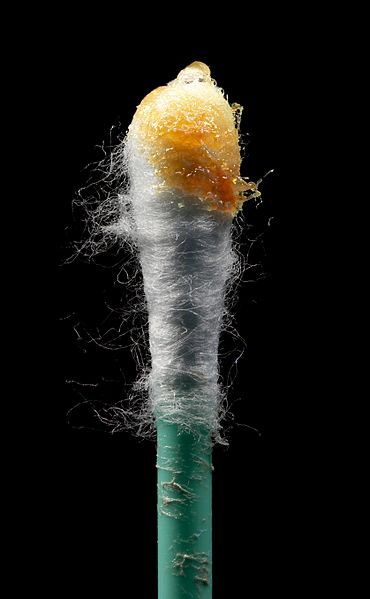
Figure 12. Wet-type human earwax on a cotton swab.
Earwax, also known by the medical term cerumen, is a yellowish, waxy substance secreted in the ear canal of humans and many other mammals. It plays a vital role in the human ear canal, assisting in cleaning and lubrication, and also provides some protection from bacteria, fungus, and insects. Excess or impacted cerumen can press against the eardrum and/or occlude the external auditory canal and impair hearing.
Production, composition, and different types
Cerumen is produced in the outer third of the cartilaginous portion of the human ear canal. It is a mixture of viscous secretions from sebaceous glands and less-viscous ones from modified apocrine sweat glands.
Two distinct genetically determined types of earwax are distinguished — the wet-type which is dominant, and the dry type which is recessive. Asians and Native Americans are more likely to have the dry type of cerumen (grey and flaky), whereas Caucasians and Africans are more likely to have the wet type (honey-brown to dark-brown and moist). Cerumen type has been used by anthropologists to track human migratory patterns, such as those of the Inuit.
The difference in cerumen type has been tracked to a single base change (an single nucleotide polymorphism) in a gene known as “ATP-binding cassette C11 gene”. In addition to affecting cerumen type, this mutation also reduces sweat production. The researchers conjecture that the reduction in sweat was beneficial to the ancestors of East Asians and Native Americans who are thought to have lived in cold climates.
Function
Cleaning. Cleaning of the ear canal occurs as a result of the “conveyor belt” process of epithelial migration, aided by jaw movement. Cells formed in the center of the tympanic membrane migrate outwards from the umbo (at a rate equivalent to that of fingernail growth) to the walls of the ear canal, and accelerate towards the entrance of the ear canal. The cerumen in the canal is also carried outwards, taking with it any dirt, dust, and particulate matter that may have gathered in the canal. Jaw movement assists this process by dislodging debris attached to the walls of the ear canal, increasing the likelihood of its extrusion.
Lubrication. Lubrication prevents desiccation and itching of the skin within the ear canal (known as asteatosis). The lubricative properties arise from the high lipid content of the sebum produced by the sebaceous glands. In wet-type cerumen at least, these lipids include cholesterol, squalene, and many long-chain fatty acids and alcohols.
Antibacterial and antifungal roles. While studies conducted up until the 1960s found little evidence supporting an antibacterial role for cerumen, more recent studies have found that cerumen provides some bactericidal protection against some strains of bacteria. Cerumen has been found to be effective in reducing the viability of a wide range of bacteria (sometimes by up to 99%), including Haemophilus influenzae, Staphylococcus aureus, and many variants of Escherichia coli. The growth of two fungi commonly present in otomycosis was also significantly inhibited by human cerumen. These antimicrobial properties are due principally to the presence of saturated fatty acids, lysozyme and, especially, to the relatively low pH of cerumen (typically around 6.1 in normal individuals).
Mammary Glands
Mammary glands are the organs that, in the female mammal, produce milk for the sustenance of the young. These exocrine glands are enlarged and modified sweat glands and are the characteristic of mammals which gave the class its name.
Structure
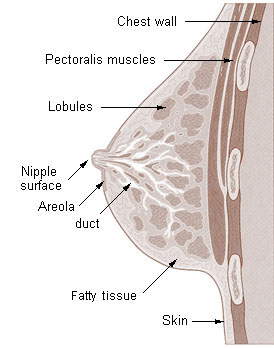
Figure 13. Cross section of the breast of a human female.
The basic components of the mammary gland are the alveoli (hollow cavities, a few millimetres large) lined with milk-secreting epithelial cells and surrounded by myoepithelial cells. These alveoli join up to form groups known as lobules, and each lobule has a lactiferous duct that drains into openings in the nipple. The myoepithelial cells can contract, similar to muscle cells, and thereby push the milk from the alveoli through the lactiferous ducts towards the nipple, where it collects in widenings (sinuses) of the ducts. A suckling baby essentially squeezes the milk out of these sinuses.
One distinguishes between a simple mammary gland, which consists of all the milk-secreting tissue leading to a single lactiferous duct, and a complex mammary gland, which consists of all the simple mammary glands serving one nipple.
Humans normally have two complex mammary glands, one in each breast, and each complex mammary gland consists of 10–20 simple glands. (The presence of more than two nipples is known as polythelia and the presence of more than two complex mammary glands as polymastia.)
Development and hormonal control
The development of mammary glands is controlled by hormones. The mammary glands exist in both sexes, but they are rudimentary until puberty when in response to ovarian hormones, they begin to develop in the female. Estrogen promotes formation, while testosterone inhibits it.
At the time of birth, the baby has lactiferous ducts but no alveoli. Little branching occurs before puberty when ovarian estrogens stimulate branching differentiation of the ducts into spherical masses of cells that will become alveoli. True secretory alveoli only develop in pregnancy, where rising levels of estrogen and progesterone cause further branching and differentiation of the duct cells, together with an increase in adipose tissue and a richer blood flow.
Colostrum is secreted in late pregnancy and for the first few days after giving birth. True milk secretion (lactation) begins a few days later due to a reduction in circulating progesterone and the presence of the hormone prolactin. The suckling of the baby causes the release of the hormone oxytocin which stimulates contraction of the myoepithelial cells.
Check Your Understanding
Answer the question(s) below to see how well you understand the topics covered in the previous section. This short quiz does not count toward your grade in the class, and you can retake it an unlimited number of times.
Use this quiz to check your understanding and decide whether to (1) study the previous section further or (2) move on to the next section.
Candela Citations
- Introduction to Support Systems. Authored by: Shelli Carter and Lumen Learning. Provided by: Lumen Learning. License: CC BY: Attribution
- Human Physiology. Provided by: Wikibooks. Located at: https://en.wikibooks.org/wiki/Human_Physiology. License: CC BY-SA: Attribution-ShareAlike
- Muscle Types Image. Provided by: OpenStax CNX. Located at: http://cnx.org/contents/185cbf87-c72e-48f5-b51e-f14f21b5eabd@10.8. Project: Biology. License: CC BY: Attribution. License Terms: Download for free at http://cnx.org/contents/185cbf87-c72e-48f5-b51e-f14f21b5eabd@10.8
- An eText of Human Anatomy and Physiology. Authored by: Dr. Bruce Forciea. Located at: http://www.bruceforciea.com/etextchapters/etexthumananatrevmay12.pdf. License: CC BY: Attribution
- Transverse Section Of Bone. Authored by: BDB. Located at: https://commons.wikimedia.org/wiki/File:Transverse_Section_Of_Bone.png. License: CC BY: Attribution
- The Skeletal System. Authored by: Crash Course. Located at: https://youtu.be/rDGqkMHPDqE. Project: Crash Course A&P. License: All Rights Reserved. License Terms: Standard YouTube License
- Illu long bone. Provided by: Cancer.gov. Located at: https://commons.wikimedia.org/wiki/File:Illu_long_bone.jpg. License: Public Domain: No Known Copyright
- Osteocyte. Provided by: Wikimedia Commons. Located at: https://commons.wikimedia.org/wiki/File:Osteocyte_2.jpg. License: Public Domain: No Known Copyright
- Human skeleton front. Authored by: LadyofHats. Located at: https://commons.wikimedia.org/wiki/File:Human_skeleton_front_en.svg. License: Public Domain: No Known Copyright