Nine vitamins are considered water soluble, including: vitamin C and all of the B vitamins (Riboflavin, Niacin, Thiamin, B6, Folate, B12, Pantothenic Acid and Biotin). In contrast to the four Fat-Soluble Vitamins, Water-soluble vitamins dissolve easily in water and, in general, are readily excreted from the body, to the degree that urinary output is a strong predictor of vitamin consumption. Because they are not as readily stored, more consistent intake is important. Many types of water-soluble vitamins are synthesized by bacteria.
7.3A: Vitamin B₁ (Thiamine)
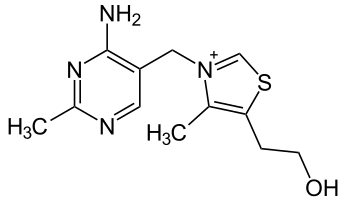
Figure 7.3A.1: Structure of thiamine
Thiamin is found in a wide variety of processed and whole foods, with edible seeds, legumes, rice and processed foods, such as breakfast cereals, having among the highest contents. Some other foods naturally rich in thiamin are corn flour, pork, pecans and spinach.
Dietary Reference Intakes
The Food and Nutrition Board of the U.S. Institute of Medicine updated Estimated Average Requirements (EARs) and Recommended Dietary Allowances (RDAs) for thiamine in 1998. The current EARs for thiamine for women and men ages 14 and up are 0.9 mg/day and 1.0 mg/day, respectively; the RDAs are 1.1 and 1.2 mg/day. RDAs are higher than EARs so as to identify amounts that will cover people with higher than average requirements. RDA for pregnancy equals 1.4 mg/day. RDA for lactation equals 1.4 mg/day. For infants up to 12 months the Adequate Intake (AI) is 0.2-0.3 mg/day. and for children ages 1–13 years the RDA increases with age from 0.5 to 0.9 mg/day.
As for safety, the Food and Nutrition Board of the U.S. Institute of Medicine sets Tolerable Upper Intake Levels (known as ULs) for vitamins and minerals when evidence is sufficient. In the case of thiamine there is no UL, as there is no human data for adverse effects from high doses. The European Food Safety Authority reviewed the same safety question and also reached the conclusion that there was not sufficient evidence to set a UL for thiamine. Collectively the EARs, RDAs and ULs are referred to as Dietary Reference Intakes.
For U.S. food and dietary supplement labeling purposes the amount in a serving is expressed as a percent of Daily Value (%DV). For thiamine labeling purposes 100% of the Daily Value was 1.5 mg, but as of May 2016 it has been revised to 1.2 mg. A table of the pre-change adult Daily Values is provided at Reference Daily Intake. Food and supplement companies have until July 28, 2018 to comply with the change.
Antagonists
Thiamine in foods can be degraded in a variety of ways. Sulfites, which are added to foods usually as a preservative, will attack thiamine at the methylene bridge in the structure, cleaving the pyrimidine ring from the thiazole ring. The rate of this reaction is increased under acidic conditions. Thiamine is degraded by thermolabile thiaminases (present in raw fish and shellfish). Some thiaminases are produced by bacteria. Bacterial thiaminases are cell surface enzymes that must dissociate from the membrane before being activated; the dissociation can occur in ruminants under acidotic conditions. Rumen bacteria also reduce sulfate to sulfite, therefore high dietary intakes of sulfate can have thiamine-antagonistic activities.
Plant thiamine antagonists are heat-stable and occur as both the ortho- and para-hydroxyphenols. Some examples of these antagonists are caffeic acid, chlorogenic acid, and tannic acid. These compounds interact with the thiamine to oxidize the thiazole ring, thus rendering it unable to be absorbed. Two flavonoids, quercetin and rutin, have also been implicated as thiamine antagonists.
Absorption
Thiamine is released by the action of phosphatase and pyrophosphatase in the upper small intestine. At low concentrations, the process is carrier-mediated, and, at higher concentrations, absorption occurs via passive diffusion. Active transport is greatest in the jejunum and ileum; but, active transport can be inhibited by alcohol consumption and by folic deficiency. Decline in thiamine absorption occurs at intakes above 5 mg/day. The cells of the intestinal mucosa have thiamine pyrophosphokinase activity, but it is unclear as to whether the enzyme is linked to active absorption. The majority of thiamine present in the intestine is in the pyrophosphorylated form ThDP, but when thiamine arrives on the serosal side of the intestine it is often in the free form. The uptake of thiamine by the mucosal cell is likely coupled in some way to its phosphorylation/dephosphorylation. On the serosal side of the intestine, evidence has shown that discharge of the vitamin by those cells is dependent on Na+-dependent ATPase.
Uptake of thiamine by cells of the blood and other tissues occurs via active transport and passive diffusion. The brain requires much more thiamine than other tissues of the body. Much of ingested thiamine never reaches the brain because of passive diffusion and the blood–brain barrier. About 80% of intracellular thiamine is phosphorylated and most is bound to proteins. In some tissues, thiamine uptake and secretion appears to be mediated by a soluble thiamine transporter that is dependent on Na+ and a transcellular proton gradient.
Human storage of thiamine is about 25 to 30 mg, with the greatest concentrations in skeletal muscle, heart, brain, liver, and kidneys. ThMP and free (unphosphorylated) thiamine is present in plasma, milk, cerebrospinal fluid, and, it is presumed, all extracellular fluids. Unlike the highly phosphorylated forms of thiamine, ThMP and free thiamine are capable of crossing cell membranes. Thiamine contents in human tissues are less than those of other species.
Contributors
- Wikipedia. Content is copyrighted under a CC-BY-SA 4.0 license.
7.3B: Vitamin B₂ (Riboflavin)
Riboflavin, also known as vitamin B2, is a vitamin found in food and used as a dietary supplement. As a supplement it is used to prevent and treat riboflavin deficiency and prevent migraines. It may be given by mouth or injection. It is nearly always well tolerated. Normal doses are safe during pregnancy. Riboflavin is in the vitamin B group. It is required by the body for cellular respiration. Food sources include eggs, green vegetables, milk, and meat. Riboflavin was discovered in 1920, isolated in 1933, and first made in 1935. It is on the World Health Organization’s List of Essential Medicines, the most effective and safe medicines needed in a health system. Riboflavin is available as a generic medication and over the counter. In the United States a month of supplements costs less than 25 USD. Some countries require its addition to grains.

A solution of riboflavin. Image used with permission (CC BY-SA 4.0; PatríciaR)
Riboflavin has been used in several clinical and therapeutic situations. For over 30 years, riboflavin supplements have been used as part of the phototherapy treatment of neonatal jaundice. The light used to irradiate the infants breaks down not only bilirubin, the toxin causing the jaundice, but also the naturally occurring riboflavin within the infant’s blood, so extra supplementation is necessary.
Riboflavin functions as a coenzyme, meaning that it is required for enzymes (proteins) to perform normal physiological actions. Specifically, the active forms of riboflavin flavin mononucleotide (FMN) and flavin adenine dinucleotide (FAD) function as cofactors for a variety of flavoproteine enzyme reactions:
- Flavoproteins of electron transport chain, including FMN in Complex I and FAD in Complex II
- FAD is required for the production of pyridoxic acid from pyridoxal (vitamin B6) by pyridoxine 5′-phosphate oxidase
- The primary coenzyme form of vitamin B6 (pyridoxal phosphate) is FMN dependent
- Oxidation of pyruvate, α-ketoglutarate, and branched-chain amino acids requires FAD in the shared E3 portion of their respective dehydrogenase complexes
- Fatty acyl CoA dehydrogenase requires FAD in fatty acid oxidation
- FAD is required to convert retinol (vitamin A) to retinoic acid via cytosolic retinal dehydrogenase
- Synthesis of an active form of folate (5-methyltetrahydrofolate) from 5,10-methylenetetrahydrofolate by Methylenetetrahydrofolate reductase is FADH2 dependent
- FAD is required to convert tryptophan to niacin (vitamin B3)
- Reduction of the oxidized form of glutathione (GSSG) to its reduced form (GSH) by Glutathione reductase is FAD dependent
For the molecular mechanism of action see main articles Flavin mononucleotide (FMN) and flavin adenine dinucleotide (FAD)
Dietary Sources
Food and beverages that provide riboflavin without fortification are milk, cheese, eggs, leaf vegetables, liver, kidneys, legumes, mushrooms, and almonds. The milling of cereals results in considerable loss (up to 60%) of vitamin B2, so white flour is enriched in some countries such as US by addition of the vitamin. The enrichment of bread and ready-to-eat breakfast cereals contributes significantly to the dietary supply of vitamin B2. Polished rice is not usually enriched, because the vitamin’s yellow color would make the rice visually unacceptable to the major rice-consumption populations. However, most of the flavin content of whole brown rice is retained if the rice is steamed (parboiled) prior to milling. This process drives the flavins in the germ and aleurone layers into the endosperm. Free riboflavin is naturally present in foods along with protein-bound FMN and FAD. Bovine milk contains mainly free riboflavin, with a minor contribution from FMN and FAD. In whole milk, 14% of the flavins are bound noncovalently to specific proteins. Egg white and egg yolk contain specialized riboflavin-binding proteins, which are required for storage of free riboflavin in the egg for use by the developing embryo.
Dietary Reference Intake
In humans, there is no evidence for riboflavin toxicity produced by excessive intakes, in part because it has lower water solubility than other B vitamins, because absorption becomes less efficient as doses increase, and because what excess is absorbed is excreted via the kidneys into urine. Even when 400 mg of riboflavin per day was given orally to subjects in one study for three months to investigate the efficacy of riboflavin in the prevention of migraine headache, no short-term side effects were reported. Although toxic doses can be administered by injection, any excess at nutritionally relevant doses is excreted in the urine, imparting a bright yellow color when in large quantities.
The Food and Nutrition Board of the U.S. Institute of Medicine sets Tolerable Upper Intake Levels (known as ULs) for vitamins and minerals when evidence is sufficient. In the case of riboflavin there is no UL, as there is no human data for adverse effects from high doses. The European Food Safety Authority reviewed the same safety question and also reached the conclusion that there was not sufficient evidence to set a UL for riboflavin.
The Food and Nutrition Board of the U.S. Institute of Medicine updated Estimated Average Requirements (EARs) and Recommended Dietary Allowances (RDAs) in 1998. The current EARs for riboflavin for women and men ages 14 and up are 0.9 mg/day and 1.1 mg/day, respectively; the RDAs are 1.1 and 1.3 mg/day. RDAs are higher than EARs so as to identify amounts that will cover people with higher than average requirements. RDA for pregnancy equals 1.4 mg/day. RDA for lactation equals 1.6 mg/day. For infants up to 12 months the Adequate Intake (AI) is 0.3-0.4 mg/day and for children ages 1–13 years the RDA increases with age from 0.5 to 0.9 mg/day. Collectively the EARs, RDAs and ULs (see Toxicity) are referred to as Dietary Reference Intakes.[18][26]
For U.S. food and dietary supplement labeling purposes the amount in a serving is expressed as a percent of Daily Value (%DV). For riboflavin labeling purposes 100% of the Daily Value was 1.7 mg, but as of May 2016 it has been revised to 1.3 mg. A table of the pre-change adult Daily Values is provided at Reference Daily Intake. Food and supplement companies have until July 2018 to comply with the change.
RIBOFLAVIN DEFICIENCY
Mild deficiencies can exceed 50% of the population in third world countries and in refugee situations. Deficiency is uncommon in the United States and in other countries that have wheat flour, bread, pasta, corn meal or rice enrichment regulations. In the U.S., starting in the 1940s, flour, corn meal and rice have been fortified with B vitamins as a means of restoring some of what is lost in milling, bleaching and other processing. For adults 20 and older, average intake from food and beverages is 1.8 mg/day for women and 2.5 mg/day for men. An estimated 23% consume a riboflavin-containing dietary supplement that provides on average 10 mg. The U.S. Department of Health and Human Services conducts National Health and Nutrition Examination Survey every two years and reports food results in a series of reports referred to as “What We Eat In America.” From NHANES 2011–2012, the latest for which data has been reported, estimates are that 8% of women and 3% of men consume less than the RDA. When compared to the lower Estimated Average Requirements, fewer than 3% do not achieve the EAR level. However, anyone choosing a gluten-free or low gluten diet should as a precaution take a multi-vitamin/mineral dietary supplement which provides 100% DV for riboflavin and other B vitamins.
Riboflavin deficiency (also called ariboflavinosis) results in stomatitis including painful red tongue with sore throat, chapped and fissured lips (cheilosis), and inflammation of the corners of the mouth (angular stomatitis). There can be oily scaly skin rashes on the scrotum, vulva, philtrum of the lip, or the nasolabial folds. The eyes can become itchy, watery, bloodshot and sensitive to light. Due to interference with iron absorption, even mild to moderate riboflavin deficiency results in an anemia with normal cell size and normal hemoglobin content (i.e. normochromic normocytic anemia). This is distinct from anemia caused by deficiency of folic acid (B9) or cyanocobalamin (B12), which causes anemia with large blood cells (megaloblastic anemia). Deficiency of riboflavin during pregnancy can result in birth defects including congenital heart defects and limb deformities.
The stomatitis symptoms are similar to those seen in pellagra, which is caused by niacin (B3) deficiency. Therefore, riboflavin deficiency is sometimes called “pellagra sine pellagra” (pellagra without pellagra), because it causes stomatitis but not widespread peripheral skin lesions characteristic of niacin deficiency.
7.3C: Vitamin B₃ (Niacin)
Niacin, also known as vitamin B3 or nicotinic acid, is an organic compound with the formula C6H5NO2C6H5NO2 and, depending on the definition used, one of the 20 to 80 essential human nutrients. Pharmaceutical and supplemental niacin are primarily used to treat hypercholesterolemia (high cholesterol) and pellagra (niacin deficiency). Insufficient niacin in the diet can cause nausea, skin and mouth lesions, anemia, headaches, and tiredness. The lack of niacin may also be observed in pandemic deficiency disease, which is caused by a lack of five crucial vitamins (niacin, vitamin C, thiamin, vitamin D, and vitamin A) and is usually found in areas of widespread poverty and malnutrition. Niacin is provided in the diet from a variety of whole and processed foods, with highest contents in fortified packaged foods and meat from various animal sources.
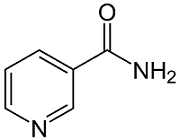
Structure of nicotinamide (niacinamide).
Niacin supplementation has not been found useful for decreasing the risk of cardiovascular disease in those already on a statin, but appears to be effective in those not taking a statin.[Although niacin and nicotinamide are identical in their vitamin activity, nicotinamide does not have the same pharmacological effects (lipid modifying effects) as niacin. Nicotinamide does not reduce cholesterol or cause flushing. As the precursor for NAD and NADP, niacin is also involved in DNA repair.
Dietary Reference Intake
The Food and Nutrition Board of the U.S. Institute of Medicine updated Estimated Average Requirements (EARs) and Recommended Dietary Allowances (RDAs) for niacin in 1998. The current EARs for niacin for women and men ages 14 and up are 11 mg/day and 12 mg/day, respectively; the RDAs are 14 and 16 mg/day, respectively. RDAs are higher than EARs so as to identify amounts that will cover people with higher than average requirements. RDA for pregnancy equals 18 mg/day. RDA for lactation equals 17 mg/day. For infants up to 12 months the Adequate Intake (AI) is 2–4 mg/day. and for children ages 1–13 years the RDA increases with age from 6 to 12 mg/day. As for safety, the Food and Nutrition Board also sets Tolerable Upper Intake Levels (known as ULs) for vitamins and minerals when evidence is sufficient. In the case of niacin the UL is set at 35 mg/day.[11] The European Food Safety Authority reviewed the same safety question and set its UL at 10 mg/day.[12] Safety issues are presented at length in the Side Effects section. Collectively the EARs, RDAs, AIs and ULs are referred to as Dietary Reference Intakes.
For U.S. food and dietary supplement labeling purposes, the amount in a serving is expressed as a percent of Daily Value (%DV). For niacin labeling purposes, 100% of the Daily Value was 20 mg, but as of May 2016 it has been revised to 16 mg. A table of the pre-change adult Daily Values is provided at Reference Daily Intake. Food and supplement companies have until July 28, 2018, to comply with the change.
Dietary Sources
Niacin is found in a variety of whole and processed foods, including fortified packaged foods, meat from various animal sources, seafoods, and spices. Among whole food sources with the highest niacin content per 100 grams:
Meats
- cooked skipjack tuna, 18.8 mg
- cooked light meat turkey, 11.8 mg
- cooked, lean ground pork, 11.1 mg
- cooked venison, 10.8 mg
- cooked, lean veal, 8.0 mg
Plant foods and spices
- sesame seed flour, 12.5 mg
- ground ginger, 9.6 mg
- dried tarragon, 9.0 mg
- dried, green sweet peppers, 7.4 mg
- grilled portabella mushrooms, 6.2 mg
- roasted sunflower seeds, 4.1 mg
- dehydrated apricots, 3.6 mg
- baked potato, 3.1 mg
Fortified breakfast cereals have among the highest niacin contents (more than 20 mg per 100 grams).[13] Whole grain flours, such as from wheat, rice, barley or corn, and pasta have niacin contents in a range of 3–10 mg per 100 grams.
Deficiencies
Between 1906 and 1940 more than 3 million Americans were affected by pellagra with more than 100,000 deaths. Dr. Joseph Goldberger was assigned to study pellagra by the Surgeon General of the United States and produced good results. In the late 1930s, studies by Dr. Tom Spies, Marion Blankenhorn, and Clark Cooper established that niacin cured pellagra in humans. The disease was greatly reduced as a result.
At present, niacin deficiency is sometimes seen in developed countries, and it is usually apparent in conditions of poverty, malnutrition, and chronic alcoholism. It also tends to occur in areas where people eat maize (corn) as a staple food, since it is the only grain low in digestible niacin. A cooking technique called nixtamalization increases the bioavailability of niacin during maize meal/flour production.

A man with pellegra, which is caused by a chronic lack of vitamin B3 in the diet
Mild niacin deficiency has been shown to slow metabolism, causing decreased tolerance to cold. Severe deficiency of niacin in the diet causes the disease pellagra, which is characterized by diarrhea, dermatitis, and dementia, as well as Casal’s necklace lesions on the lower neck, hyperpigmentation, thickening of the skin, inflammation of the mouth and tongue, digestive disturbances, amnesia, delirium, and eventually death, if left untreated. Common psychiatric symptoms of niacin deficiency include irritability, poor concentration, anxiety, fatigue, restlessness, apathy, and depression. Studies have indicated that, in patients with alcoholic pellagra, niacin deficiency may be an important factor influencing both the onset and severity of this condition. Patients with alcoholism typically experience increased intestinal permeability, leading to negative health outcomes.
7.3D: Vitamin B₅ (Pantothenic acid)
Pantothenic acid, also called vitamin B5, is a water-soluble vitamin. Pantothenic acid is an essential nutrient. Animals require pantothenic acid to synthesize coenzyme-A (CoA), as well as to synthesize and metabolize proteins, carbohydrates, and fats. Pantothenic acid is the amide between pantoic acid and β-alanine. Its name derives from the Greek pantothen, meaning “from everywhere”, and small quantities of pantothenic acid are found in nearly every food, with high amounts in fortified whole-grain cereals, egg yolks, liver and dried mushrooms. It is commonly found as its alcohol analog, the provitamin panthenol (pantothenol), and as calcium pantothenate.
-Pantothenic_acid_Formula_V.1.svg.png?revision=1)
Skeletal formula of (R)-pantothenic acid
Dietary Sources
Content of pantothenic acid varies among manufactured and natural foods, especially fortified ready-to-eat cereals, infant formulas, energy bars and dried foods. Major food sources of pantothenic acid are dried mushrooms, liver, dried egg yolks and sunflower seeds. Whole grains are another good source of the vitamin, but milling removes much of the pantothenic acid, as it is found in the outer layers of whole grains. In animal feeds, the most important sources are alfalfa, cereal, fish meal, peanut meal, molasses, mushrooms, rice, wheat bran, and yeasts.
Dietary Reference Intake
The Food and Nutrition Board of the U.S. Institute of Medicine updated Estimated Average Requirements (EARs) and Recommended Dietary Allowances (RDAs) for B vitamins in 1998. At that time there was not sufficient information to establish EARs and RDAs for pantothenic acid. In instances such as this, the Board sets Adequate Intakes (AIs), with the understanding that at some later date, AIs will be replaced by more exact information. As for safety, the FNB sets Tolerable Upper Intake Levels (known as ULs) for vitamins and minerals when evidence is sufficient. In the case of pantothenic acid there is no UL, as there is insufficient human data to identify adverse effects from high doses. The European Food Safety Authority reviewed the same safety question and also reached the conclusion that there was not sufficient evidence to set a UL for pantothenic acid. Collectively the EARs, RDAs and ULs are referred to as Dietary Reference Intakes.
For U.S. food and dietary supplement labeling purposes the amount in a serving is expressed as a percent of Daily Value (%DV). For pantothenic acid labeling purposes 100% of the Daily Value was 10 mg, but as of May 2016 it has been revised to 5 mg. Food and supplement companies have until July 2018 to comply with the change.
Age group | Age | Adequate intake |
---|---|---|
Infants | 0–6 months | 1.7 mg |
Infants | 7–12 months | 1.8 mg |
Children | 1–3 years | 2 mg |
Children | 4–8 years | 3 mg |
Children | 9–13 years | 4 mg |
Adult men and women | 14+ years | 5 mg |
Pregnant women | (vs. 5) | 6 mg |
Breastfeeding women | (vs. 5) | 7 mg |
Toxicity of pantothenic acid is unlikely. In fact, no Tolerable Upper Level Intake (UL) has been established for the vitamin. Large doses of the vitamin, when ingested, have no reported side effects and massive doses (e.g., 10 g/day) may only yield mild intestinal distress, and diarrhea at worst. It has been suggested, however, that high doses of pantothenic acid might worsen panic attacks in those with panic disorder by prolonging the duration until adrenal exhaustion. Pantothenic acid, at a human equivalent dose within the range of common supplementation, was shown to induce adrenal hyper-responsiveness to stress stimulation. There are also no adverse reactions known following parenteral (injected) or topical (skin) applications of the vitamin.
Absorption
When found in foods, most pantothenic acid is in the form of CoA or acyl carrier protein (ACP). For the intestinal cells to absorb this vitamin, it must be converted into free pantothenic acid. Within the lumen of the intestine, CoA and ACP are hydrolyzed into 4′-phosphopantetheine. The 4′-phosphopantetheine is then dephosphorylated into pantetheine. Pantetheinase, an intestinal enzyme, then hydrolyzes pantetheine into free pantothenic acid. Free pantothenic acid is absorbed into intestinal cells via a saturable, sodium-dependent active transport system. At high levels of intake, when this mechanism is saturated, some pantothenic acid may also be absorbed via passive diffusion. As intake increases 10-fold, however, absorption rate decreases to 10%.
Deficiency
Pantothenic acid deficiency is exceptionally rare and has not been thoroughly studied. In the few cases where deficiency has been seen (victims of starvation and limited volunteer trials), nearly all symptoms can be reversed with the return of pantothenic acid. Symptoms of deficiency are similar to other vitamin B deficiencies. There is impaired energy production, due to low CoA levels, which could cause symptoms of irritability, fatigue, and apathy.Acetylcholine synthesis is also impaired; therefore, neurological symptoms can also appear in deficiency; they include numbness, paresthesia, and muscle cramps. Deficiency in pantothenic acid can also cause hypoglycemia, or an increased sensitivity to insulin. Insulin receptors are acylated with palmitic acid when they do not want to bind with insulin. Therefore, more insulin will bind to receptors when acylation decreases, causing hypoglycemia. Additional symptoms could include restlessness, malaise, sleep disturbances, nausea, vomiting, and abdominal cramps. In a few rare circumstances, more serious (but reversible) conditions have been seen, such as adrenal insufficiency and hepatic encephalopathy.
7.3E: Vitamin B₆ (Pyridoxine)
Vitamin B6 refers to a group of chemically similar compounds which can be interconverted in biological systems. Vitamin B6 is part of the vitamin B group of essential nutrients. Its active form, pyridoxal 5′-phosphate, serves as a coenzyme in some 100 enzyme reactions in amino acid, glucose, and lipid metabolism. Several forms
- Pyridoxine 5′-phosphate (P5P)
- Pyridoxal (PL)
- Pyridoxal 5′-phosphate (PLP), the metabolically active form (sold as P-5-P vitamin supplement)
- Pyridoxamine (PM)
- Pyridoxamine 5′-phosphate (PMP)
- 4-Pyridoxic acid (PA), the catabolite which is excreted in urine
- Pyritinol, a semi-synthetic derivative of pyridoxine, where two pyridoxine moieties are bound by a disulfide bridge.
All forms except pyridoxic acid and pyritinol can be interconverted. Absorbed pyridoxamine is converted to PMP by pyridoxal kinase, which is further converted to PLP by pyridoxamine-phosphate transaminase or pyridoxine 5′-phosphate oxidase which also catalyzes the conversion of PNP to PLP. Pyridoxine 5′-phosphate oxidase is dependent on flavin mononucleotide (FMN) as a cofactor which is produced from riboflavin (vitamin B2) i.e. in this biochemical pathway, dietary vitamin B6 cannot be used without vitamin B2.

Pyridoxine and Pyridoxamine
PLP, the metabolically active form of vitamin B6, is involved in many aspects of mcronutrient metabolism, neurotransmitter synthesis, histamine synthesis, hemoglobin synthesis and function, and gene expression. PLP generally serves as a coenzyme (cofactor) for many reactions including decarboxylation, transamination, racemization, elimination, replacement, and beta-group interconversion. The liver is the site for vitamin B6 metabolism.Amino acid metabolism PLP is a cofactor in the biosynthesis of five important neurotransmitters: serotonin, dopamine, epinephrine, norepinephrine, and gamma-aminobutyric acid (GABA). PLP is also involved in the synthesis of histamine.
Dietary Sources
Vitamin B6 is widely distributed in foods in both its free and bound forms. Cooking, storage, and processing losses of vitamin B6 vary and in some foods may be more than 50%, depending on the form of vitamin present in the food. Plant foods lose the least during processing, as they contain mostly pyridoxine, which is far more stable than the pyridoxal or pyridoxamine found in animal foods. For example, milk can lose 30–70% of its vitamin B6 content when dried. Vitamin B6 is found in the germ and aleurone layer of grains, and milling results in the reduction of this vitamin in white flour. The heating that occurs before most freezing and canning processes are other methods that may result in the loss of vitamin B6 in foods.
Foods that contain large amounts of vitamin B6 include:
- fortified breakfast cereals
- pork
- turkey
- beef
- bananas
- chickpeas
- potatoes
- pistachios
Dietary Reference Intake
The Food and Nutrition Board (FNB) of the U.S. Institute of Medicine updated Estimated Average Requirements (EARs) and Recommended Dietary Allowances (RDAs) for vitamin B6 in 1998. The current EARs for vitamin B6 for women and men ages 14 and up increase with age from 1.0 to 1.3 mg/day and from 1.1 to 1.4 mg/day, respectively; the RDAs increase with age from 1.2 to 1.5 and from 1.3 to 1.7 mg/day, respectively. RDAs are higher than EARs so as to identify amounts that will cover people with higher than average requirements. RDA for pregnancy equals 1.9 mg/day. RDA for lactation equals 2.0 mg/day. For infants up to 12 months the Adequate Intake (AI) is 0.1–0.3 mg/day. and for children ages 1–13 years the RDA increases with age from 0.5 to 1.0 mg/day. As for safety, the FNB sets Tolerable Upper Intake Levels (known as ULs) for vitamins and minerals when evidence is sufficient. In the case of vitamin B6 the UL is set at 100 mg/day. The European Food Safety Authority reviewed the same safety question and set its UL at 25 mg/day. Safety issues are presented at length in the Toxicity section.
For U.S. food and dietary supplement labeling purposes the amount in a serving is expressed as a percent of daily value (%DV). For vitamin B6 labeling purposes 100% of the Daily Value was 2.0 mg, but as of May 2016 it has been revised to 1.7 mg. Food and supplement companies have until July 28, 2018 to comply with the change.
Absorption and excretion
Vitamin B6 is absorbed in the jejunum and ileum by passive diffusion. With the capacity for absorption being so great, animals are able to absorb quantities much greater than necessary for physiological demands. The absorption of pyridoxal phosphate and pyridoxamine phosphate involves their dephosphorylation catalyzed by a membrane-bound alkaline phosphatase. Those products and nonphosphorylated forms in the digestive tract are absorbed by diffusion, which is driven by trapping of the vitamin as 5′-phosphates through the action of phosphorylation (by a pyridoxal kinase) in the jejunal mucosa. The trapped pyridoxine and pyridoxamine are oxidized to pyridoxal phosphate in the tissue.
The products of vitamin B6 metabolism are excreted in the urine, the major product of which is 4-pyridoxic acid. An estimated 40–60% of ingested vitamin B6 is oxidized to 4-pyridoxic acid. Several studies have shown that 4-pyridoxic acid is undetectable in the urine of vitamin B6-deficient subjects, making it a useful clinical marker to assess the vitamin B6 status of an individual. Other products of vitamin B6metabolism excreted in the urine when high doses of the vitamin have been given include pyridoxal, pyridoxamine, and pyridoxine and their phosphates. A small amount of vitamin B6 is also excreted in the feces.
Deficiencies
The classic clinical syndrome for vitamin B6 deficiency is a seborrhoeic dermatitis-like eruption, atrophic glossitis with ulceration, angular cheilitis, conjunctivitis, intertrigo, and neurologic symptoms of somnolence, confusion, and neuropathy (due to impaired sphingosine synthesis) and sideroblastic anemia (due to impaired heme synthesis).
Less severe cases present with metabolic disease associated with insufficient activities of the coenzyme PLP. The most prominent of the lesions is due to impaired tryptophan–niacin conversion. This can be detected based on urinary excretion of xanthurenic acid after an oral tryptophan load. Vitamin B6 deficiency can also result in impaired transsulfuration of methionine to cysteine. The PLP-dependent transaminases and glycogen phosphorylase provide the vitamin with its role in gluconeogenesis, so deprivation of vitamin B6 results in impaired glucose tolerance.
A deficiency of vitamin B6 alone is relatively uncommon and often occurs in association with other vitamins of the B complex. The elderly and alcoholics have an increased risk of vitamin B6 deficiency, as well as other micronutrient deficiencies. Evidence exists for decreased levels of vitamin B6 in women with type 1 diabetes and in patients with systemic inflammation, liver disease, rheumatoid arthritis, and those infected with HIV.
7.3F: Vitamin B₇ (Biotin)
Biotin is a water-soluble B-vitamin, also called vitamin B7 and formerly known as vitamin H or coenzyme R.[2] It is composed of a ureido ring fused with a tetrahydrothiophene ring. A valeric acid substituent is attached to one of the carbon atoms of the tetrahydrothiophene ring. Biotin is a coenzyme for carboxylase enzymes, involved in the synthesis of fatty acids, isoleucine, and valine, and in gluconeogenesis. Biotin deficiency can be caused by inadequate dietary intake or inheritance of one or more inborn genetic disorders that affect biotin metabolism. Subclinical deficiency can cause mild symptoms, such as hair thinning or skin rash typically on the face. Neonatal screening for biotinidase deficiency began in the United States in 1984 and today many countries test for this disorder at birth. Individuals born prior to 1984 are unlikely to have been screened, thus the true prevalence of the disorder is unknown.

Skeletal formula of biotin
Biotin is necessary for cell growth, the production of fatty acids, and the metabolism of fats and amino acids. Biotin assists in various metabolic reactions involving the transfer of carbon dioxide. It may also be helpful in maintaining a steady blood sugar level. Biotin is often recommended as a dietary supplement for strengthening hair and nails, though scientific data supporting this outcome are weak. Nevertheless, biotin is found in many cosmetics and health products for the hair and skin.
Dietary Reference Intake
The Food and Nutrition Board of the U.S. Institute of Medicine updated Estimated Average Requirements (EARs) and Recommended Dietary Allowances (RDAs) for B vitamins in 1998. At that time there was not sufficient information to establish EARs and RDAs for biotin. In instances such as this, the Board sets Adequate Intakes (AIs), with the understanding that at some later date, AIs will be replaced by more exact information. The current AI for adults ages 19 and up is 30 μg/day. AI for pregnancy is 30 μg/day. AI for lactation is 35 μg/day. For infants up to 12 months the AI is 5-6 μg/day For children ages 1–18 years the AI increases with age from 8 to 25 μg/day.
As for safety, the FNB sets Tolerable Upper Intake Levels (known as ULs) for vitamins and minerals when evidence is sufficient. In the case of biotin there is no UL, as there is insufficient human data to identify adverse effects from high doses. The European Food Safety Authority reviewed the same safety question and also reached the conclusion that there was not sufficient evidence to set a UL for biotin.
For U.S. food and dietary supplement labeling purposes the amount in a serving is expressed as a percent of Daily Value (%DV). For biotin labeling purposes 100% of the Daily Value was 300 μg, but as of May 2016 it has been revised to 30 μg to bring it into agreement with the AI. Food and supplement companies have until July 2018 to comply with the change.
Dietary Sources
Biotin is synthesized by intestinal bacteria, but there is a lack of good quality studies about how much biotin they provide. Biotin is stable at room temperature and isn’t destroyed by cooking. Some of the best sources are (content per 100 grams):
- Brewer’s yeast: 188.8 mcg
- Soybeans: 179.4 mcg
- Beef liver: 113.3 mcg
- Butter: 94.3 mcg
- Split peas: 77.7 mcg
- Sunflower seeds: 66 mcg
- Green peas/lentils: 40 mcg
- Peanuts/walnuts: 37.5 mcg
- Pecans: 27.75 mcg
- Eggs: 18.9 mcg
Egg whites contain a protein (avidin) that blocks the absorption of biotin, so people who regularly consume a large number of eggs may become biotin-deficient. The dietary biotin intake in Western populations has been estimated to be 35 to 70 micrograms per day (143–287 nmol per day). Biotin is also available in dietary supplements in which a dose of 30 micrograms meets 100% of the Daily Value for adults.
Deficiency
Biotin deficiency is rare. The amounts needed are small, a wide range of foods contain biotin, and intestinal bacteria synthesize biotin, which is then absorbed by the host animal. For that reason, statutory agencies in many countries, for example the USA and Australia, have not formally established a recommended daily intake of biotin. Instead, an Adequate Intake (AI) is identified based on the theory that average intake meets needs. A number of rare metabolic disorders exist in which an individual’s metabolism of biotin is abnormal.
Biotin deficiency typically occurs from dietary absence of the vitamin. Consuming raw egg whites over months may result in biotin deficiency. Deficiency can be addressed with nutritional supplementation. Deficiency symptoms include:
- Brittle and thin fingernails
- Hair loss (alopecia)
- Conjunctivitis
- Dermatitis in the form of a scaly, red rash around the eyes, nose, mouth, and genital area.
- Neurological symptoms in adults, such as depression, lethargy, hallucination, and numbness and tingling of the extremities
The neurological and psychological symptoms can occur with only mild deficiencies. Dermatitis, conjunctivitis, and hair loss will generally occur only when deficiency becomes more severe. Individuals with hereditary disorders of biotin deficiency have evidence of impaired immune system function, including increased susceptibility to bacterial and fungal infections.
Pregnant women tend to have a high risk of biotin deficiency. Nearly half of pregnant women have abnormal increases of 3-hydroxyisovaleric acid, which reflects reduced status of biotin. Several studies have reported this possible biotin deficiency during the pregnancy may cause infants’ congenital malformations, such as cleft palate. Mice fed with dried raw egg to induce biotin deficiency during the gestation resulted in up to 100% incidence of the infants’ malnourishment. Infants and embryos are more sensitive to the biotin deficiency. Therefore, even a mild level of the mother’s biotin deficiency that does not reach the appearance of physiological deficiency signs may cause a serious consequence in the infants.
7.3G: Vitamin B₉ (Folic acid)

Skeletal formula
There are no common side effects. It is not known whether high doses over a long period of time are of concern. There are concerns that large amounts of folic acid might hide vitamin B12 deficiency. It is essential for the body to make DNA, RNA, and metabolise amino acids which are required for cell division.[8] As humans cannot make folic acid, it is required from the diet, making it an essential vitamin.
Not consuming enough folate can lead to folate deficiency. This may result in a type of anemia in which low numbers of large red blood cells occur. Symptoms may include feeling tired, heart palpitations, shortness of breath, open sores on the tongue, and changes in the color of the skin or hair. Deficiency in children may develop within a month of poor dietary intake.[13] In adults normal total body folate is between 10,000–30,000 micrograms (µg) with blood levels of greater than 7 nmol/L (3 ng/mL).[8]
Folic acid was discovered between 1931 and 1943.[14] It is on the World Health Organization’s List of Essential Medicines, the most effective and safe medicines needed in a health system.[15] The wholesale cost of supplements in the developing world is between 0.001 and 0.005 USD per dose as of 2014.[16]The term “folic” is from the Latin word folium, which means leaf. Folates occur naturally in many foods especially dark green leafy vegetables and liver.[8]
Pregnancy and Fertility
Folic acid intake during pregnancy has been linked to a lessened risk of neural tube defects. Likewise, a meta-analysis of folic acid supplementation during pregnancy reported a 28% lower risk of newborn congenital heart defects. The United States Preventive Services Task Force recommends folic acid supplementation for all women able to become pregnant.
Devakamar reviewed long-term outcomes for live births that did not involve neural tube defects and fortification with folic acid did not have an impact on childhood survival, growth, body composition, or cognitive outcomes. Prenatal supplementation did not appear to reduce the risk of pre-term births. And there does not appear to be a correlation between maternal folic acid supplementation and an increased risk for asthma in the child.
Folate is necessary for fertility in both men and women. It contributes to spermatogenesis. Therefore, it is necessary to receive sufficient amounts through the diet to avoid subfertility. Also, polymorphisms in genes of enzymes involved in folate metabolism could be one reason for fertility complications in some women with unexplained infertility.
Heart Disease, Stroke and Cancer
Taking folic acid over years reduced the risk of cardiovascular disease by 4%, where another study found it did not affect cardiovascular disease, even while reducing homocysteine levels.
Long-term supplementation with folic acid reduced the risk of stroke by 10%, which may be due to the role folate plays in regulating homocysteine concentration.The reviews indicate the risk of stroke appears to be reduced only in some individuals, but a definite recommendation regarding supplementation beyond the current RDA has not been established for stroke prevention. Asian populations had greater protection against stroke with folate supplementation than did European or North American subjects.
Observed stroke reduction is consistent with the reduction in pulse pressure produced by folate supplementation of 5 mg per day, since hypertension is a key risk factor for stroke. Folic supplements are inexpensive and relatively safe to use, which is why stroke or hyperhomocysteinemia patients are encouraged to consume daily B vitamins including folic acid.
Studies on folic acid intake from food and folate supplementation with regards to cancer risk are based on the adequacy of chronic intake. Chronically insufficient intake of folic acid (below the recommended level of 400 micrograms per day) may increase the risk of colorectal, breast, ovarian, pancreas, brain, lung, cervical, and prostate cancers. Other studies showed that excessive dietary supplementation with synthetic folate may increase the risk of certain cancers, in particular prostate. A 2017 review found no relationship between taking folate supplements and cancer risk.
Dietary Reference Intake
Because of the difference in bioavailability between supplemented folic acid and the different forms of folate found in food, the dietary folate equivalent (DFE) system was established. One DFE is defined as 1 μg of dietary folate, or 0.6 μg of folic acid supplement.
Age | Infants (AI) | Infants (UL) | Adults (RDA) | Adults (UL) | Pregnant women (RDA) | Pregnant women (UL) | Lactating women (RDA) | Lactating women (UL) |
---|---|---|---|---|---|---|---|---|
0–6 months | 65 | None set | – | – | – | – | – | – |
7–12 months | 80 | None set | – | – | – | – | – | – |
1–3 years | – | – | 150 | 300 | – | – | – | – |
4–8 years | – | – | 200 | 400 | – | – | – | – |
9–13 years | – | – | 300 | 600 | – | – | – | – |
14–18 | – | – | 400 | 800 | 600 | 800 | 500 | 800 |
19+ | – | – | 400 | 1000 | 600 | 1000 | 500 | 1000 |
For U.S. food and dietary supplement labeling purposes the amount in a serving is expressed as a percent of Daily Value (%DV). For folic acid labeling purposes 100% of the Daily Value was 400 μg. As of the May 2016 update it was kept unchanged at 400 μg. A table of the pre-change adult Daily Values is provided at Reference Daily Intake. Food and supplement companies have until 28 July 2018 to comply with the change.
Dietary Sources
Folate naturally occurs in a wide variety of foods, including vegetables (particularly dark green leafy vegetables), fruits and fruit juices, nuts, beans, peas, dairy products, poultry and meat, eggs, seafood, grains, and some beers. Avocado, beetroot, spinach, liver, yeast, asparagus, and Brussels sprouts are among the foods with the highest levels of folate. Folic acid is added to grain products in many countries, and in these countries, fortified products make up a significant source of the population’s folic acid intake. Because of the difference in bioavailability between supplemented folic acid and the different forms of folate found in food, the dietary folate equivalent (DFE) system was established. 1 DFE is defined as 1 μg of dietary folate, or 0.6 μg of folic acid supplement. This is reduced to 0.5 μg of folic acid if the supplement is taken on an empty stomach. bFolate naturally found in food is susceptible to high heat and ultraviolet light, and is soluble in water. It is heat-labile in acidic environments and may also be subject to oxidation. Some meal replacement products do not meet the folate requirements as specified by the RDAs.
FOOD FORTIFICATION
Folic acid fortification is a process where folic acid is added to flour with the intention of promoting public health through increasing blood folate levels in the populace. In the USA, food is fortified with folic acid, only one of the many naturally occurring forms of folate, and a substance contributing only a minor amount to the folates in natural foods. Since the discovery of the link between insufficient folic acid and neural tube defects, governments and health organizations worldwide have made recommendations concerning folic acid supplementation for women intending to become pregnant.
Fortification is controversial, with issues having been raised concerning individual liberty, as well as the health concerns described in the Toxicity section above. In the USA, there is concern that the federal government mandates fortification, but does not provide monitoring of potential undesirable effects of fortification. 76 countries worldwide (inclduing the USA) require mandatory folic acid fortification of at least one major cereal grain, with nearly all fortifying at least wheat flour, according to November 2013 data from the Flour Fortification Initiative.
Folate Deficiency
Folate deficiency can be caused by unhealthy diets that do not include enough fruits and vegetables, diseases in which folic acid is not well absorbed in the digestive system (such as Crohn’s disease or celiac disease), some genetic disorders that affect levels of folate, and certain medicines (such as phenytoin, sulfasalazine, or trimethoprim-sulfamethoxazole). Folate deficiency is accelerated by alcohol consumption.
Folate deficiency may lead to glossitis, diarrhea, depression, confusion, anemia, and fetal neural tube defects and brain defects (during pregnancy). Other symptoms include fatigue, gray hair, mouth sores, poor growth, and swollen tongue. Folate deficiency is diagnosed by analyzing CBC and plasma vitamin B12 and folate levels. CBC may indicate megaloblastic anemia but this could also be a sign of vitamin B12 deficiency. A serum folate of 3 μg/L or lower indicates deficiency. Serum folate level reflects folate status but erythrocyte folate level better reflects tissue stores after intake. Serum folate reacts more rapidly to folate intake than erythrocyte folate. An erythrocyte folate level of 140 μg/L or lower indicates inadequate folate status. Increased homocysteine level suggests tissue folate deficiency but homocysteine is also affected by vitamin B12 and vitamin B6, renal function, and genetics.
7.3H: Vitamin B₁₂ (Cobalamin)
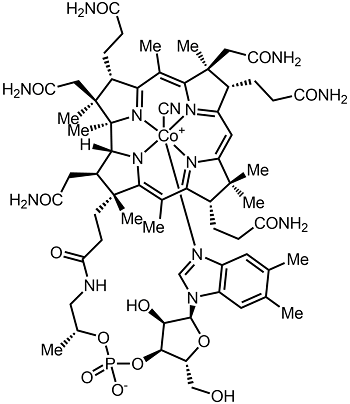
Chemical structure of cyanocobalamin. Image used with permission (CC BY-SA 4.0; Alsosaid1987).
Vitamin B12 consists of a class of chemically related compounds (vitamers), all of which show pharmacological activity. It contains the biochemically rare element cobalt (chemical symbol Co) positioned in the center of a planar tetra-pyrrole ring called a corrin ring. The vitamer is produced by bacteria as hydroxocobalamin, but conversion between different forms of the vitamin occurs in the body after consumption.
Dietary Reference Intake
The Food and Nutrition Board of the U.S. Institute of Medicine (IOM) updated Estimated Average Requirements (EARs) and Recommended Dietary Allowances (RDAs) for vitamin B12 in 1998. The current EAR for vitamin B12 for women and men ages 14 and up is 2.0 μg/day; the RDA is 2.4 μg/day. RDAs are higher than EARs so as to identify amounts that will cover people with higher than average requirements. RDA for pregnancy equals 2.6 μg/day. RDA for lactation equals 2.8 μg/day. For infants up to 12 months the Adequate Intake (AI) is 0.4-0.5 μg/day. and for children ages 1–13 years the RDA increases with age from 0.9 to 1.8 μg/day. Because 10 to 30 percent of older people may be unable to effectively absorb vitamin B12 naturally occurring in foods, it is advisable for those older than 50 years to meet their RDA mainly by consuming foods fortified with vitamin B12 or a supplement containing vitamin B12.
For U.S. food and dietary supplement labeling purposes the amount in a serving is expressed as a percent of Daily Value (%DV). For vitamin B12 labeling purposes 100% of the Daily Value was 6.0 μg, but as of May 2016 has been revised downward to 2.4 μg.
Deficiency
Vitamin B12 deficiency can potentially cause severe and irreversible damage, especially to the brain and nervous system. At levels only slightly lower than normal, a range of symptoms such as fatigue, lethargy, depression, poor memory, breathlessness, headaches, and pale skin, among others, may be experienced, especially in elderly people (over age 60) who produce less stomach acid as they age, thereby increasing their probability of B12deficiencies.
Vitamin B12 deficiency is most commonly caused by low intakes, but can also result from malabsorption, certain intestinal disorders, low presence of binding proteins, and use of certain medications. Vitamin B12 is rare from plant sources, so vegetarians are most likely to suffer from vitamin B12 deficiency. Infants are at a higher risk of vitamin B12 deficiency if they were born to vegetarian mothers. The elderly who have diets with limited meat or animal products are vulnerable populations as well. Vitamin B12 deficiency may occur in between 40% to 80% of the vegetarian population.
Vitamin B12 is a co-substrate of various cell reactions involved in methylation synthesis of nucleic acid and neurotransmitters. Synthesis of the trimonoamine neurotransmitters can enhance the effects of a traditional antidepressant. The intracellular concentrations of vitamin B12 can be inferred through the total plasma concentration of homocysteine, which can be converted to methionine through an enzymatic reaction that uses 5-methyltetrahydrofolate as the methyl donor group. Consequently, the plasma concentration of homocysteine falls as the intracellular concentration of vitamin B12 rises. The active metabolite of vitamin B12 is required for the methylation of homocysteine in the production of methionine, which is involved in a number of biochemical processes including the monoamine neurotransmitters metabolism. Thus, a deficiency in vitamin B12 may impact the production and function of those neurotransmitters.
Dietary Sources
Foods having rich content of vitamin B12 include clams, organ meats (especially liver) from lamb, veal, beef, and turkey, fish eggs, mackerel, and crab meat. B12 is synthesized by some gut bacteria in humans and other animals, but humans cannot absorb the B12 made in their guts, as it is made in the colon which is too far from the small intestine, where absorption of B12 occurs.
Animals store vitamin B12 in liver and muscle and some pass the vitamin into their eggs and milk; meat, liver, eggs and milk are therefore sources of the vitamin for other animals, including people. For humans, the bioavailability from eggs is less than 9%, compared to 40% to 60% from fish, fowl and meat. Insects are also a source of B12. Foods fortified with B12 are also dietary sources of the vitamin. Foods for which B12-fortified versions are widely available include breakfast cereals, soy products, energy bars, and nutritional yeast.
Vitamin B12 is an ingredient in multi-vitamin pills and in some countries used to enrich grain-based foods such as bread and pasta. In the U.S. non-prescription products can be purchased providing up to 5000 µg/serving, and it is a common ingredient in energy drinks and energy shots, usually at many times the recommended dietary allowance of B12. The vitamin can also be a prescription product via injection or other means.
Absorption and Distribution
Methyl-B12 is absorbed by two processes. The first is an intestinal mechanism using intrinsic factor through which 1-2 micrograms can be absorbed every few hours. The second is a diffusion process by which approximately 1% of the remainder is absorbed. The human physiology of vitamin B12 is complex, and therefore is prone to mishaps leading to vitamin B12 deficiency. Protein-bound vitamin B12must be released from the proteins by the action of digestive proteases in both the stomach and small intestine.
Absorption of food vitamin B12 thus requires an intact and functioning stomach, exocrine pancreas, intrinsic factor, and small bowel. Problems with any one of these organs makes a vitamin B12 deficiency possible. Individuals who lack intrinsic factor have a decreased ability to absorb B12. The total amount of vitamin B12 stored in body is about 2–5 mg in adults. Around 50% of this is stored in the liver. Approximately 0.1% of this is lost per day by secretions into the gut, as not all these secretions are reabsorbed. Bile is the main form of B12 excretion; most of the B12 secreted in the bile is recycled via enterohepatic circulation. Excess B12 beyond the blood’s binding capacity is typically excreted in urine. Owing to the extremely efficient enterohepatic circulation of B12, the liver can store 3 to 5 years’ worth of vitamin B12; therefore, nutritional deficiency of this vitamin is rare. How fast B12levels change depends on the balance between how much B12 is obtained from the diet, how much is secreted and how much is absorbed. B12 deficiency may arise in a year if initial stores are low and genetic factors unfavorable, or may not appear for decades. In infants, B12 deficiency can appear much more quickly.
7.3I: Vitamin C (Ascorbic acid)
Vitamin C (ascorbic acid and L-ascorbic acid) is a vitamin found in food and used as a dietary supplement. As a supplement it is used to treat and prevent scurvy. Evidence does not support use in the general population for the prevention of the common cold. It may be taken by mouth or by injection. It is generally well tolerated. Large doses may cause gastrointestinal upset, headache, trouble sleeping, and flushing of the skin. Normal doses are safe during pregnancy. Vitamin C is an essential nutrient involved in the repair of tissue. Foods that contain vitamin C include citrus fruit, tomatoes, and potatoes.
Vitamin C was discovered in 1912, isolated in 1928, and first made in 1933. It is on the World Health Organization’s List of Essential Medicines, the most effective and safe medicines needed in a health system. Vitamin C is available as a generic medication and over the counter. In 2015, the wholesale cost in the developing world was about 0.003 to 0.007 USD per tablet. In some countries, ascorbic acid may be added to foods such as breakfast cereal.
Medical uses
A 2012 Cochrane review found no effect of vitamin C supplementation on overall mortality.
- Scurvy: Although rare in modern times, scurvy and its associated destabilization of collagen, connective tissue, and bone can be prevented by adequate vitamin C intake.
- Cancer prevention: A 2014 review found that, “Currently, the use of high-dose IV vitamin C [as an anticancer agent] cannot be recommended outside of a clinical trial.” A 2013 Cochrane review found no evidence that vitamin C supplementation reduces the risk of lung cancer in healthy or high risk (smokers and asbestos-exposed) people. A 2014 meta-analysis found that vitamin C intake might protect against lung cancer risk. A second meta-analysis found no effect on the risk of prostate cancer. Two meta-analyses evaluated the effect of vitamin C supplementation on the risk of colorectal cancer. One found a weak association between vitamin C consumption and reduced risk, and the other found no effect of supplementation. A 2011 meta-analysis failed to find support for the prevention of breast cancer with vitamin C supplementation,but a second study concluded that vitamin C may be associated with increased survival in those already diagnosed.
- Cardiovascular disease: A 2013 meta-analysis found no evidence that vitamin C supplementation reduces the risk of myocardial infarction, stroke, cardiovascular mortality, or all-cause mortality. However, a second analysis found an inverse relationship between circulating vitamin C levels or dietary vitamin C and the risk of stroke. A meta-analysis of 44 clinical trials has shown a significant positive effect of vitamin C on endothelial function when taken at doses greater than 500 mg per day. The researchers noted that the effect of vitamin C supplementation appeared to be dependent on health status, with stronger effects in those at higher cardiovascular disease risk.
- Chronic diseases: A 2010 review found no role for vitamin C supplementation in the treatment of rheumatoid arthritis. Studies examining the effects of vitamin C intake on the risk of Alzheimer’s disease have reached conflicting conclusions. Maintaining a healthy dietary intake is probably more important than supplementation for achieving any potential benefit. Vitamin C supplementation above the RDA has been used in trials to study a potential effect on preventing and slowing the progression of age-related cataract. However, no significant effects were found from the research.
- Common cold: Vitamin C’s effect on the common cold has been extensively researched. It has not been shown effective in prevention or treatment of the common cold, except in limited circumstances (specifically, individuals exercising vigorously in cold environments). Routine vitamin C supplementation does not reduce the incidence or severity of the common cold in the general population, though it may reduce the duration of illness.
Possible
As vitamin C enhances iron absorption, iron poisoning can become an issue to people with rare iron overload disorders, such as haemochromatosis. A genetic condition that results in inadequate levels of the enzyme glucose-6-phosphate dehydrogenase (G6PD) can cause sufferers to develop hemolytic anemia after ingesting specific oxidizing substances, such as very large dosages of vitamin C.
There is a longstanding belief among the mainstream medical community that vitamin C causes kidney stones, which is based on little science. Although recent studies have found a relationship, a clear link between excess ascorbic acid intake and kidney stone formation has not been generally established. Some case reports exist for a link between patients with oxalate deposits and a history of high-dose vitamin C usage.
In a study conducted on rats, during the first month of pregnancy, high doses of vitamin C may suppress the production of progesterone from the corpus luteum. Progesterone, necessary for the maintenance of a pregnancy, is produced by the corpus luteum for the first few weeks, until the placenta is developed enough to produce its own source. By blocking this function of the corpus luteum, high doses of vitamin C (1000+ mg) are theorized to induce an early miscarriage. In a group of spontaneously aborting women at the end of the first trimester, the mean values of vitamin C were significantly higher in the aborting group. However, the authors do state: ‘This could not be interpreted as an evidence of causal association.’ However, in a previous study of 79 women with threatened, previous spontaneous, or habitual abortion, Javert and Stander (1943) had 91% success with 33 patients who received vitamin C together with bioflavonoids and Vitamin K (only three abortions), whereas all of the 46 patients who did not receive the vitamins aborted.
A study in rats and humans suggested that adding Vitamin C supplements to an exercise training program lowered the expected effect of training on VO2 Max. Although the results in humans were not statistically significant, this study is often cited as evidence that high doses of Vitamin C have an adverse effect on exercise performance. In rats, it was shown that the additional Vitamin C resulted in lowered mitochondria production. Since rats are able to produce all of their needed Vitamin C, however, it is questionable whether they offer a relevant model of human physiological processes in this regard.
Biological Significance
Vitamin C is an essential nutrient for certain animals including humans. Vitamin C describes several vitamers that have vitamin C activity in animals, including ascorbic acid and its salts, and some oxidized forms of the molecule like dehydroascorbic acid. Ascorbate and ascorbic acid are both naturally present in the body when either of these is introduced into cells, since the forms interconvert according to pH. Vitamin C is a cofactor in at least eight enzymatic reactions, including several collagen synthesis reactions that, when dysfunctional, cause the most severe symptoms of scurvy. In animals, these reactions are especially important in wound-healing and in preventing bleeding from capillaries. Ascorbate also acts as an antioxidant, protecting against oxidative stress.
The biological role of ascorbate is to act as a reducing agent, donating electrons to various enzymatic and a few non-enzymatic reactions. The one- and two-electron oxidized forms of vitamin C, semidehydroascorbic acid and dehydroascorbic acid, respectively, can be reduced in the body by glutathione and NADPH-dependent enzymatic mechanisms. The presence of glutathione in cells and extracellular fluids helps maintain ascorbate in a reduced state.
In humans, vitamin C is essential to a healthy diet as well as being a highly effective antioxidant, acting to lessen oxidative stress; a substrate for ascorbate peroxidase in plants (APX is plant specific enzyme); and an enzyme cofactor for the biosynthesis of many important biochemicals. Vitamin C acts as an electron donor for important enzymes.
Ascorbate is required for a range of essential metabolic reactions in all animals and plants. It is made internally by almost all organisms; the main exceptions are most bats, all guinea pigs, capybaras, and the Haplorrhini (one of the two major primate suborders, consisting of tarsiers, monkeys, and humans and other apes). Ascorbate is also not synthesized by many species of birds and fish. All species that do not synthesize ascorbate require it in the diet.
DEFICIENCY
Scurvy is an avitaminosis resulting from lack of vitamin C, since without this vitamin, the synthesized collagen is too unstable to perform its function. Scurvy leads to the formation of brown spots on the skin, spongy gums, and bleeding from all mucous membranes. The spots are most abundant on the thighs and legs, and a person with the ailment looks pale, feels depressed, and is partially immobilized. In advanced scurvy there are open, suppurating wounds and loss of teeth and, eventually, death. The human body can store only a certain amount of vitamin C, and so the body stores are depleted if fresh supplies are not consumed. The time frame for onset of symptoms of scurvy in unstressed adults on a completely vitamin C free diet, however, may range from one month to more than six months, depending on previous loading of vitamin C.
Western societies generally consume far more than sufficient vitamin C to prevent scurvy. In 2004, a Canadian Community health survey reported that Canadians of 19 years and above have intakes of vitamin C from food of 133 mg/d for males and 120 mg/d for females; these are higher than the RDA recommendations.
Notable human dietary studies of experimentally induced scurvy have been conducted on conscientious objectors during WWII in Britain and on Iowa state prisoners in the late 1960s to the 1980s. These studies both found that all obvious symptoms of scurvy previously induced by an experimental scorbutic diet with extremely low vitamin C content could be completely reversed by additional vitamin C supplementation of only 10 mg a day. In these experiments, there was no clinical difference noted between men given 70 mg vitamin C per day (which produced blood level of vitamin C of about 0.55 mg/dl, about 1/3 of tissue saturation levels) and those given 10 mg per day. Men in the prison study developed the first signs of scurvy about 4 weeks after starting the vitamin C-free diet, whereas in the British study, six to eight months were required, possibly due to the pre-loading of this group with a 70 mg/day supplement for six weeks before the scorbutic diet was fed.
Men in both studies on a diet devoid, or nearly devoid, of vitamin C had blood levels of vitamin C too low to be accurately measured when they developed signs of scurvy, and in the Iowa study, at this time were estimated (by labeled vitamin C dilution) to have a body pool of less than 300 mg, with daily turnover of only 2.5 mg/day, implying an instantaneous half-life of 83 days by this time (elimination constant of 4 months).
Absorption, transport, and excretion
Ascorbic acid is absorbed in the body by both active transport and simple diffusion. Sodium-Dependent Active Transport—Sodium-Ascorbate Co-Transporters (SVCTs) and Hexose transporters (GLUTs)—are the two transporters required for absorption. SVCT1 and SVCT2 import the reduced form of ascorbate across plasma membrane.GLUT1 and GLUT3 are the two glucose transporters, and transfer only the dehydroascorbic acid form of Vitamin C.[82] Although dehydroascorbic acid is absorbed in higher rate than ascorbate, the amount of dehydroascorbic acid found in plasma and tissues under normal conditions is low, as cells rapidly reduce dehydroascorbic acid to ascorbate. Thus, SVCTs appear to be the predominant system for vitamin C transport in the body.
SVCT2 is involved in vitamin C transport in almost every tissue,[81] the notable exception being red blood cells, which lose SVCT proteins during maturation.[85] “SVCT2 knockout” animals genetically engineered to lack this functional gene, die shortly after birth,[86] suggesting that SVCT2-mediated vitamin C transport is necessary for life.
With regular intake the absorption rate varies between 70 and 95%. However, the degree of absorption decreases as intake increases. At high intake (1.25 g), fractional human absorption of ascorbic acid may be as low as 33%; at low intake (<200 mg) the absorption rate can reach up to 98%.
Ascorbate concentrations over the renal re-absorption threshold pass freely into the urine and are excreted. At high dietary doses (corresponding to several hundred mg/day in humans) ascorbate is accumulated in the body until the plasma levels reach the renal resorption threshold, which is about 1.5 mg/dL in men and 1.3 mg/dL in women. Concentrations in the plasma larger than this value (thought to represent body saturation) are rapidly excreted in the urine with a half-life of about 30 minutes. Concentrations less than this threshold amount are actively retained by the kidneys, and the excretion half-life for the remainder of the vitamin C store in the body thus increases greatly, with the half-life lengthening as the body stores are depleted. This half-life rises until it is as long as 83 days by the onset of the first symptoms of scurvy.[88]
Although the body’s maximal store of vitamin C is largely determined by the renal threshold for blood, there are many tissues that maintain vitamin C concentrations far higher than in blood. Biological tissues that accumulate over 100 times the level in blood plasma of vitamin C are the adrenal glands, pituitary, thymus, corpus luteum, and retina.[89] Those with 10 to 50 times the concentration present in blood plasma include the brain, spleen, lung, testicle, lymph nodes, liver, thyroid, small intestinal mucosa, leukocytes, pancreas, kidney, and salivary glands.
Ascorbic acid can be oxidized (broken down) in the human body by the enzyme L-ascorbate oxidase. Ascorbate that is not directly excreted in the urine as a result of body saturation or destroyed in other body metabolism is oxidized by this enzyme and removed.
Immune system
Vitamin C is found in high concentrations in immune cells, and is consumed quickly during infections. It is not certain how vitamin C interacts with the immune system; it has been hypothesized to modulate the activities of phagocytes, the production of cytokines and lymphocytes, and the number of cell adhesion molecules in monocytes.[101]
Daily Requirements
The North American Dietary Reference Intake recommends 90 milligrams per day for adult men, 75 mg/day for adult women, and no more than 2 grams (2,000 milligrams) per day. A balanced diet without supplementation usually contains enough vitamin C to prevent scurvy in an average healthy adult, while those who smoke tobacco or are under stress require slightly more.
Recommended Dietary Allowance (adult male) | 90 mg per day |
Recommended Dietary Allowance (adult female) | 75 mg per day |
Recommended Dietary Allowance (pregnancy) | 85 mg per day |
Recommended Dietary Allowance (lactation) | 120 mg per day |
Tolerable Upper Intake Level (adult male) | 2,000 mg per day |
Tolerable Upper Intake Level (adult female) | 2,000 mg per day |
Recommended intake
Recommendations for vitamin C intake have been set by various national agencies:
- 40 milligrams per day or 280 milligrams per week taken all at once: the United Kingdom’s Food Standards Agency
- 40 milligrams per day as per the recommendations of India’s National Institute of Nutrition, Hyderabad
- 45 milligrams per day 300 milligrams per week: the World Health Organization
- 80 milligrams per day: the European Commission’s Council on nutrition labeling
- 90 mg/day (males) and 75 mg/day (females): Health Canada 2007
- 90 mg/day (males) and 75 mg/day (females): United States’ National Academy of Sciences.
- 100 milligrams per day: Japan’s National Institute of Health and Nutrition.
For U.S. food and dietary supplement labeling purposes the amount in a serving is expressed as a percent of Daily Value (%DV). For vitamin C labeling purposes 100% of the Daily Value was 60 mg, but as of May 2016 it has been revised to 90 mg. A table of the pre-change adult Daily Values is provided at Reference Daily Intake. Food and supplement companies have until July 28, 2018 to comply with the change.
Dietary Sources

Rose hips are a particularly rich source of vitamin C. Image used with permission (CC BY-SA 3.0; en:User:MPF)
The richest natural sources are fruits and vegetables. Vitamin C is the most widely taken nutritional supplement and is available in a variety of forms, including tablets, drink mixes, and in capsules. Vitamin C is absorbed by the intestines using a sodium-ion dependent channel. It is transported through the intestine via both glucose-sensitive and glucose-insensitive mechanisms. The presence of large quantities of sugar either in the intestines or in the blood can slow absorption.
While plants are generally a good source of vitamin C, the amount in foods of plant origin depends on the precise variety of the plant, soil condition, climate where it grew, length of time since it was picked, storage conditions, and method of preparation. The following table is approximate and shows the relative abundance in different raw plant sources. As some plants were analyzed fresh while others were dried (thus, artifactually increasing concentration of individual constituents like vitamin C), the data are subject to potential variation and difficulties for comparison. The amount is given in milligrams per 100 grams of fruit or vegetable and is a rounded average from multiple authoritative sources:
Plant source | Amount (mg / 100g) |
---|---|
Kakadu plum | 1000–5300 |
Camu Camu | 2800 |
Acerola | 1677 |
Seabuckthorn | 695 |
Indian gooseberry | 445 |
Rose hip | 426 |
Baobab | 400 |
Chili pepper (green) | 244 |
Guava (common, raw) | 228.3 |
Blackcurrant | 200 |
Red pepper | 190 |
Chili pepper (red) | 144 |
Parsley | 130 |
Kiwifruit | 90 |
Broccoli | 90 |
Loganberry | 80 |
Redcurrant | 80 |
Brussels sprouts | 80 |
Wolfberry (Goji) | 73 † |
Lychee | 70 |
Persimmon (native, raw) | 66.0 |
Cloudberry | 60 |
Elderberry | 60 |
† average of 3 sources; dried
Contributors
- Wikipedia. Content is copyrighted under a CC-BY-SA 4.0 license.
Candela Citations
- Water Soluble Vitamins. Authored by: Medical LibreTexts Contributors. Provided by: LibreTexts. Located at: https://med.libretexts.org/Courses/Sacramento_City_College/SCC%3A_Nutri_300_(Coppola)/Chapters/07%3A_Vitamins/7.3%3A_Water_Soluble_Vitamins. License: CC BY-NC-SA: Attribution-NonCommercial-ShareAlike